Postmortem coronal brain section showing diffuse axonal injury (DAI) in the corpus callosum after TBI (from Sharp DJ, Scott G, and Leech R, 2014).
Neuroradiologists have endeavored to find white matter changes in the acute phase of mTBI, but this task has proven difficult, and even the more recent and promising MRI sequences such as gradient echo (GRE) and susceptibility-weighted imaging (SWI) likely miss many areas of DAI. Thus conventional neuroimaging cannot yet provide conclusive answers as to whether and in what way white matter changes influence the progression from concussion to CTE. However, intriguing new data in the chronic phase of TBI have found that white matter damage may actually progress in subsequent years while gray matter injury remains stable (Bigler, 2013). For example, the longest follow-up MRI study of severe TBI to date has shown that the corpus callosum continues to lose volume at 8 years postinjury while the hippocampus does not (Tomaiuolo et al., 2012). One component of this selective white matter injury may be ongoing inflammation, as reactive microglia in association with white matter degeneration have been documented as long as 18 years after moderate or severe TBI (Johnson et al., 2013).
Diffusion tensor imaging (DTI) may hold the most promise in the study of long-term effects of TBI (Shenton et al., 2012). Recent DTI studies extending to 4 years after TBI have shown volume loss in multiple association tracts and the corpus callosum, but not gray matter (Farbota et al., 2012). Similarly, data have been presented to support the role of DAI within white matter as a contributor to subcortical volume loss (Leunissen et al., 2014). Other studies have shown that diffuse white matter injury is present in nondemented older athletes who had sports-related concussions, and that cognitive deficits are associated with these abnormalities (Tremblay et al., 2014). The onset of mood disorder after TBI was highlighted by a recent DTI study of retired professional football players documenting a correlation between microstructural abnormalities of frontal white matter tracts and depressive symptoms (Strain et al., 2013). DTI has also been used to investigate blast injury, and veterans with a history of blast injury had widespread reduction in white matter fractional anisotropy compared to those who had not sustained this form of injury (Taber et al., 2015). In this study, some blast-exposed subjects had had no symptoms of concussion, implying that blast exposure may induce subconcussive injury (Taber et al., 2015). A similar conclusion was drawn from a controlled prospective study of high school hockey and football players, who were found to have white matter changes at the end of the season that could be attributed to subconcussive blows and not to concussion (Bazarian et al., 2014).
It is clear that the older literature on dementia pugilistica finds a close counterpart in the more recent writings on CTE. Both conditions are progressive dementing disorders that share many clinical and neuropathological features (Roberts, 1969; Corsellis, Bruton, and Freeman-Browne, 1973; McKee et al., 2009; McKee et al., 2013; McKee et al., 2015). The neuropathological hallmark of each is widespread cortical tau deposition, while cortical amyloid accumulates in less than half of the reported cases (Corsellis, Bruton, and Freeman-Browne, 1973; McKee et al., 2013). In CTE, the tau neuropathology has been the basis for designating the disorder as a progressive tauopathy, and indeed the prominence of this protein at autopsy is striking. Yet this interpretation of the neuropathology of CTE may require revision when the larger picture of this disorder is taken into account.
Diffuse axonal injury and tauopathy
The assumption that the protein deposits in cortical regions are the most important neuropathological aspect of CTE appears to reflect the corticocentric bias of neuroscience. Recent reviews highlight the intracellular cortical tau aggregates making up neurofibrillary tangles as the hallmark of CTE, yet the same reviews state that axonal pathology within white matter is present in all cases (Mez, Stern, and McKee, 2013; McKee et al., 2015). From these statements it may quite logically be questioned why tau is the hallmark lesion of CTE while white matter injury is not. The ready visibility of tangles in brains where these findings are unexpected no doubt exerts a powerful influence. Moreover, the strong precedence of amyloid plaques being accepted as a core feature of the AD brain likely colors judgment as well. However, as DAI is well known in TBI, qualifies as a neuropathological feature common to all degrees of TBI severity, and regularly appears in TBI brains in the absence of tau, it is at least as plausible that white matter injury is the hallmark feature of CTE while tau is a downstream phenomenon. It is to this intriguing possibility we now turn.
To begin, tau is a normal brain protein, and is in fact concentrated in white matter areas. The majority of tau is found in axons, where it functions to stabilize microtubules and maintain normal axonal transport (Shahani and Brandt, 2002). In the brain, this phenomenon critically underlies normal axonal physiology and supports the operations of distributed neural networks. Because of its location within axons that may extend over one meter in length (Stokin and Goldstein, 2006), tau is highly vulnerable to injury. Autopsy examination after severe TBI in humans has found that, among several abnormal proteins, phosphorylated tau accumulates within injured axons and neuronal cell bodies in association with DAI (Uryu et al., 2007), and studies in mice have yielded comparable results (Tran et al., 2011). The acceleration and deceleration forces associated with DAI in mTBI are thought to exert a similar effect, causing microtubular tau to become hyperphosphorylated and aggregate within neurofibrillary tangles, where it is presumably neurotoxic (McKee et al., 2009).
All CTE cases studied thus far, from the mildest to the most severe, display DAI (McKee et al., 2013; McKee et al., 2015). While this lesion is not likely to have long-term clinical sequelae if it is mild and has been only rarely sustained, the possibility emerges that repeated DAI as a result of repetitive mTBI leads to overwhelming disruption of normal tau metabolism and subsequent progressive tauopathy with dementia. Tauopathy may therefore be a late result of the repeated white matter injury that may occur during an athlete’s or a soldier’s career. The propensity for the deposition of other proteins to be activated by TBI (Uryu et al., 2007) may depend on genetic factors that modify the relative risk of one dementia versus another. But all these proteins may be deposited as a result of upstream white matter injury in TBI.
An important question can be raised as to why single episodes of mTBI do not result in CTE. Most concussions, as reviewed earlier, resolve spontaneously, and, if they do not, psychological factors are usually found to be responsible. In this light, the interesting observation has been made that hyperphosphorylated tau may serve a protective role (Castellani et al., 2008). This aggregated protein is found in many normal brains, can exist for decades without apparent ill effect, and has both anti-oxidant and anti-apoptotic properties (Castellani et al., 2008). Perhaps the hyperphosphorylation of tau that occurs with DAI serves as a repair mechanism, assisting in the recovery process from concussion. Repetitive mTBI, in contrast, may overwhelm the capacity of this endogenous repair mechanism, and trigger a progressive tauopathy in which hyperphosphorylated tau becomes toxic to the cerebral cortex. Although more work is needed to substantiate this idea, tau may be a marker of brain repair in a manner similar to the proposed role played by amyloid in AD that was presented in Chapter 14.
A fascinating study in support of tauopathy as a sequel of DAI has recently been conducted with the brains of individuals subjected to prefrontal leucotomy. This procedure was one of several psychosurgical operations commonly performed for the treatment of severe psychiatric illness in the mid-twentieth century (Anderson and Arciniegas, 2004), and offers a unique opportunity to study the delayed effects of white matter injury. In all of the brains examined from six patients with schizophrenia who had undergone prefrontal leucotomy and then survived another 40 years, abnormal tau was selectively found in frontal cortical areas adjacent to the leucotomy lesion sites (Edgerton et al., 2014). The tau collections were found at the depths of cortical sulci in a manner closely resembling the distribution of tau in CTE (Edgerton et al., 2014). Because prefrontal leucotomy can be considered a single episode of severe DAI, the late appearance of tauopathy in cortical areas related to the white matter lesion may serve as a dramatic example of the prolonged development of tauopathy that occurs in repetitive mTBI with DAI.
A helpful clinical development in CTE has been the possibility that tau can be seen in the living brain. Although the neuroimaging of tau in the brain has lagged somewhat behind that of amyloid, efforts to develop a ligand for this protein have been initiated, and the agent T807 has recently been introduced as a means of visualizing tau when used in combination with positron emission tomography (PET) (Xia et al., 2013). If there is merit to the idea that repetitive DAI in the cerebral white matter is the triggering event for what may become CTE in vulnerable individuals, tau imaging may prove conclusive by virtue of its capacity to enable the longitudinal study of injured persons at the time of TBI and for years or decades thereafter. The spread of tau pathology from injured axons outward to the cerebral cortices and other regions could be tracked and correlated with a variety of clinical, MRI, and neuropsychological data over protracted periods. In contrast to AD, in which the onset of disease remains an unresolved conundrum, the onset of CTE can potentially be regarded as the time of the first mTBI event, and with improved case recognition and follow-up – now apparent both in the world of sports and in the military – the prospects of following the evolution of tauopathy are favorable if T807 is found to be a suitable agent, or another more suitable ligand with this capacity is identified.
As it seems likely that a only small percentage of injured persons develops CTE, and a long latency between repetitive mTBI and CTE is typical (McKee et al., 2009), many other factors may be operative in the pathogenesis of this disease. In addition to the traumatic injuries sustained, it is important to consider the influence of brain and cognitive reserve, the capacity of the white matter to repair itself, comorbid medical problems, and the role of many genetic factors, some still to be discovered. The number and the severity of blows to the head are variables that can be meaningfully studied with improved clinical evaluation. Brain and cognitive reserve may well influence the effects of TBI, and preliminary work has appeared (Randolph, Karantzoulis, and Guskiewicz, 2013). An intriguing factor may be the variable capacity of the brain to repair itself, and, following the myelin model discussed in Chapter 14, tauopathy in CTE may be another example of inadequate myelin repair (Bartzokis, 2011). Comorbid medical problems such as cardiovascular disease and alcohol abuse are likely to be important. Genetics may also explain why some brains may be better able to withstand the effects of repeated concussion with DAI. Most obviously, the potential role of the APOE ε4 genotype in hampering myelin repair may be relevant (Bartzokis, 2011), but preliminary observations of the influence of APOE status have been inconclusive thus far (McKee et al., 2009).
If DAI causes tau disruption in damaged axons and then secondary tauopathy, how does the abnormal protein become so distributed so widely across the brain? It seems increasingly clear that TBI, through the mechanism of DAI, disrupts axonal structure and function in a manner that can lead to long-term neurodegeneration in at least some individuals (Johnson, Stewart, and Smith, 2013). If the deposition of abnormal amounts of protein occurs, which in the case of CTE means tau pathology, it must be understood how this protein arrives at cortical regions to produce the more clinically apparent problems in cognition and behavior.
In this context, the issue of protein propagation has lately become a central concern of human neurobiology. Tau likely spreads via multiple mechanisms throughout the brain, as has been shown in animal models (Le et al., 2012). The idea has even been advanced that tau in CTE is actually a prion, behaving in a manner similar to pathologic proteins known to be involved in rapidly progressive dementias such as Creutzfeldt-Jakob disease (Prusiner, 2012). But the spread of tauopathy after mTBI may not be as rapid as that seen in typical prion diseases. The study of leucotomized schizophrenics discussed previously (Edgerton et al., 2014) sheds some light on this issue by suggesting that tauopathy can develop as long as 40 years after acute axonal injury.
CTE thus remains a pivotal but controversial proposal with many implications regarding the relationship of white matter injury to degenerative dementia. In light of its uncertain pathogenesis, a comprehensive recent review of CTE tellingly includes the statements that (1) axonal injury and white matter atrophy are constant features of the disease, (2) hyperphosphorylated tau is a known sequel of DAI in experimental animal studies, and (3) hyperphosphorylated tau may spread interneuronally via prion-like tau misfolding (McKee et al., 2015). It may be that the idea of white matter injury being the primum movens of CTE is in fact gaining momentum. Further investigation, ideally involving longitudinal white matter imaging, identification of tau with PET, and neuropathological study when possible, may lead to the conclusion that the dementia and tauopathy of CTE are in fact the late consequences of early and repeated DAI in individuals who were exposed to repetitive mTBI.
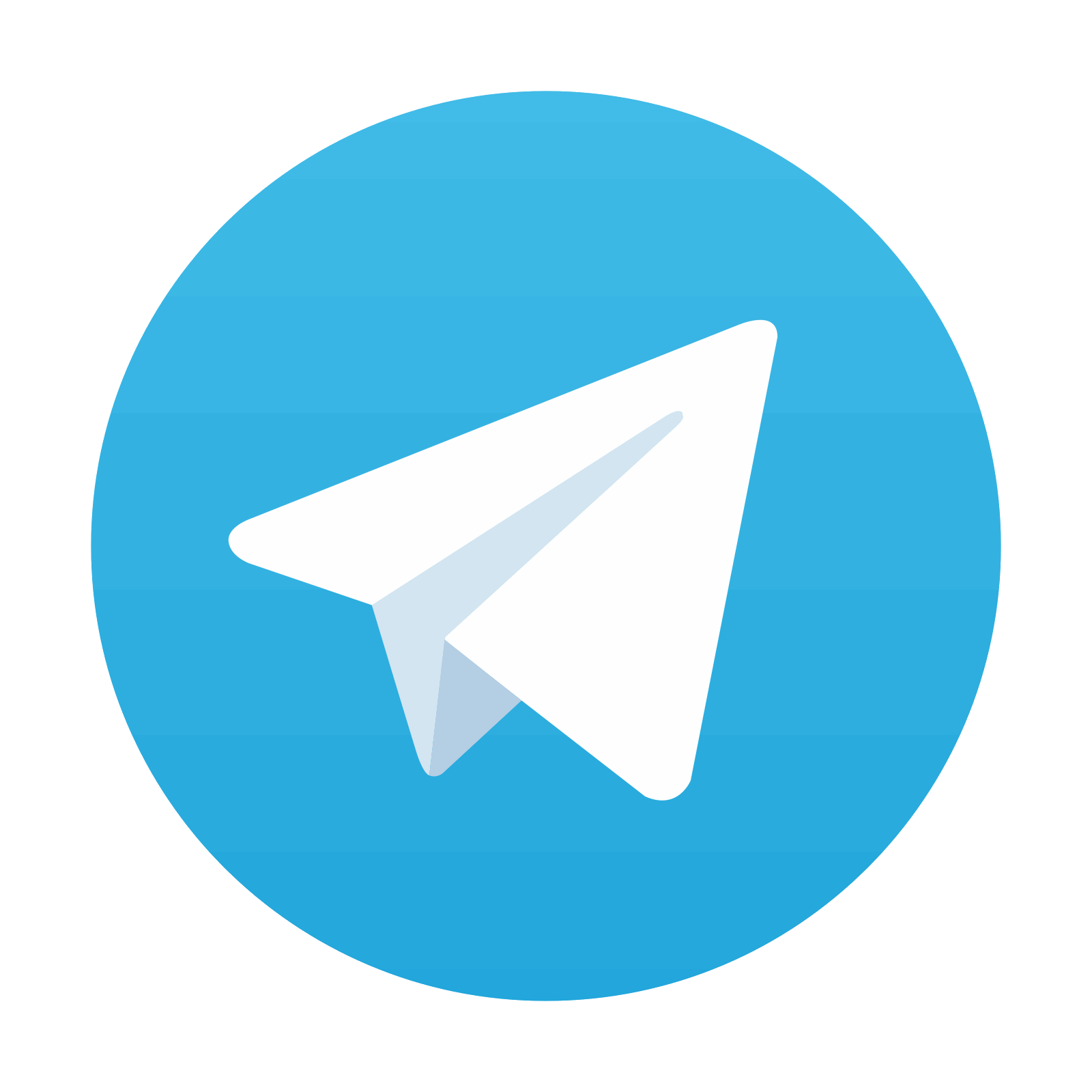
Stay updated, free articles. Join our Telegram channel

Full access? Get Clinical Tree
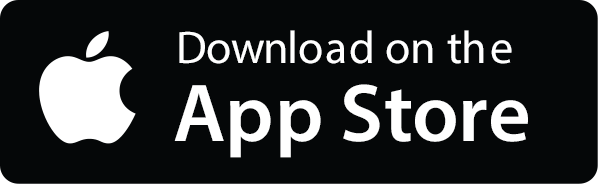
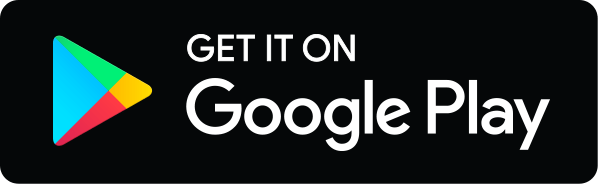