Chapter 21 Case A 65-year-old man came to medical attention with long-standing Parkinson’s disease that is intractable to medication. He also has bradykinesia and minor tremor. A subthalamic nucleus target is chosen for deep brain stimulation. Participants Deep Brain Stimulation: The Frameless Technique: Daryoush Tavanaiepour and Kathryn L. Holloway The Frame-Based Approach to Subthalamic Nucleus Deep Brain Stimulation: Tejas Sankar and Andres M. Lozano Moderator: Frame-Based vs. Frameless: Is It the Question?: Alim Louis Benabid In 1906, Clarke and Horsley1 described the first stereotactic apparatus used for animals and coined the term stereotaxic from the Greek word stereo meaning “three dimensional” and taxic meaning “an arrangement.”2 It was not until 1947, however, that Spiegel and colleagues3 introduced the first human stereotactic frame for neurosurgical procedures. Since then, there have been multiple modifications of the stereotactic frame, starting with Talairach and Riechert devices and culminating in the two most commonly used frames: the arc-based Cosman-Roberts-Wells system (CRW) system and Leksell frames.2,4–7 The stereotactic frame has been the gold standard apparatus for functional neurosurgical interventions such as lesioning or deep brain stimulation (DBS) procedures for decades.8–10 However, there are some limitations to the stereotactic frame. It is a relatively heavy device with restrictive features requiring fixation to the operating table. This can be especially problematic in patients with moderate to severe Parkinson’s disease undergoing DBS. At most institutions, patients are kept off their medications for Parkinson’s disease the night before surgery, and the stereotactic frame is placed the morning of the operation. The operative day is long, with preoperative imaging and DBS trajectory planning done on the same day, and the patient may encounter difficulties tolerating the heavy, bulky stereotactic frame after stopping medication. Furthermore, during the procedure, the frame is attached to the operating table, which can be uncomfortable and restrict the awake patient. From the physician’s perspective, the frame can prevent adequate assessment of the patient’s facial features (to examine for stimulation-induced side effects) or the observation of intraoperative stimulation efficacy in patients with axial tremor or dystonia.11,12 It also complicates management of the patient’s airway. In addition, placement of the stereo-tactic frame and patient positioning can be challenging in patients with large head diameters, cervical or thoracic kyphosis, or claustrophobia. With the development of advanced neuronavigation technology, it became possible to perform neurosurgery without the conventional stereotactic frame, and accurate localization of intracranial targets without the use of stereotactic frames has become commonplace.13,14 For procedures such as tumor resection, in which real-time feedback regarding intracranial position is helpful, image-guided surgical systems have effectively replaced stereotactic frames. Nonetheless, trajectory-based procedures, such as DBS for movement disorders, are still extensively done with a stereotactic frame15 because extreme accuracy is required for DBS surgery, as it relates directly to clinical efficacy.16–19 The benefit of real-time positional feedback is less important than the accurate delivery of the DBS lead to a well-defined target. Skin fiducial markers, as traditionally used in frameless localization, do not provide sufficient accuracy. Also, instrument holders for biopsy or other applications are not sufficiently rigid to meet the demands of true stereotactic accuracy.20 In addition, DBS procedures require a stable platform for examining the brain with micro-electrode recording and stimulation over many hours and through multiple parallel trajectories. Therefore, there was a need to develop a frameless stereotactic system specifically for DBS with accuracy and reliability equivalent to the traditional stereotactic frame but with the benefits of a frameless system. Currently, there are two frameless stereotactic systems designed specifically for DBS surgery: the STarFix (FHC, Inc., Bowdoin, ME) and the Nexframe (Medtronic, Inc., Minneapolis, MN). The STarFix system incorporates the planned trajectory into a custom-built miniature platform (surgical targeting fixture), which is attached to bone fiducial markers. Therefore, it is specific to the individual patient and procedure. A few days before surgery, the patient undergoes imaging and placement of bone fiducials. The planned trajectory and images are submitted to the company, which builds a custom device in a relatively short time (typically 3 days) with a rapid-prototyping technology. Akin to the general principles of the traditional stereotactic frame, the custom-built platform is created by translating the image space to the patient’s physical space through the bone fiducials. Furthermore, the target coordinates are referenced from the bone fiducial. Thus, the bone fiducials serve as both imaging reference points and anchors to attach the platform to the patient. This custom-built frame is sometimes referred to as a miniature frame. On the day of surgery, the completed custom-built device is attached to the patient through the previously placed bone fiducials and serves as a trajectory guide. The technical details of the STarFix system have been described previously.12,21–25 A description of the Nexframe stereotactic procedure as it is performed at our institution is outlined below. The emphasis is on the finer technical aspects, which we believe maximize the Nexframe system’s clinical accuracy. Other aspects of the procedure, such as intraoperative microelectrode recording and stimulation testing, are not discussed. The Nexframe system has been developed specifically to provide a high degree of targeting stability and accuracy during frameless DBS procedures. It depends on the surgeon’s skill rather than on the manufacturer’s quality control; it also allows the surgeon to explore more than one target at a time. The Nexframe is a skull-mounted platform linked with the Medtronic stealth neuronavigation system to provide real-time adjustment of the trajectory. The procedure is divided into two phases: preoperative and intraoperative. A few days before the surgery, preoperative imaging and bone fiducial placement are performed in an outpatient setting. We obtain a volumetric T1-weighted magnetic resonance imaging (MRI) scan with contrast to pinpoint the precise location of the intended target. The trajectory can be planned on this image at any time before the procedure. The bone fiducials are placed with sterile technique; after the administration of local anesthesia, a stab incision is made and the fiducial is inserted with a power screwdriver. The use of a power screwdriver is essential for a secure purchase of the fiducial, which will hold without loosening over several days. The fiducials are placed circumferentially around the top of the patient’s head and forehead to ensure that the predicted 1-mm accuracy of the neuronavigation sphere completely encompasses the head (Figs. 21.1, 21.2). Fiducials are not placed on the side of the head, where the patient is likely to dislodge them during sleep. Attention is also given to placing the fiducials away from the planned incision. A 0.5- to 1-mm computed tomography (CT) scan without contrast is obtained after the fiducials are placed. Next, the MRI and CT studies are loaded and merged on the Stealth neuronavigation workstation, with the CT scan as the reference image to carefully identify the center of each fiducial (Fig. 21.3). On the day of surgery, the patient does not require any further imaging or other procedures and is taken directly to the operating room. The patient is placed on a comfortable, noninvasive headrest with a cervical collar restraint (Passive Head Rest, Medtronic) (Figs. 21.1, 21.4, 21.5). The collar is kept on only during the incision and bur-hole placement, while the patient is sedated and more likely to move unexpectedly. The patient is prepared and draped with a transparent drape, which facilitates interaction with the patient, the operating room staff, and the anesthesiology and neurology team (Fig. 21.6). We also ensure that the drape encompasses all the fiducials so that they can be registered under sterile conditions (Fig. 21.7). The bur hole is made with a self-stopping cranial perforator and is widened laterally, as there is a tendency for the cannula to enter on the lateral edge of the bur hole (Fig. 21.8). The Stimloc device is then attached to the skull, followed by the Nexframe base, which is secured to the skull with the powered screwdriver to ensure stability (Fig. 21.9). If the target is the globus pallidus, a 1- to 2-mm thick Silastic sheet is placed under the lateral side of the Nexframe base, before attachment, to make alignment easier. The attachment of the Nexframe system is verified by ensuring that the Nexframe base and head move as one unit. The reference arc is then attached to the platform (Fig. 21.7). Fig. 21.2 The predicted 1-mm error sphere completely encompasses the head, and the registration error is less than 0.5 mm. During registration, we ensure that the geometry error for both the Nexprobe and reference arc is less than 0.2 mm (Fig. 21.10) by adjusting the neuronavigation camera into optimal range, moving the operating room lights off the field, and verifying that the spheres are snapped on properly and are clean. When we register the fiducials, the Nexprobe is placed in-axis with the fiducial, and the geometry error is verified again to be less than 0.2 mm (Figs. 21.7, 21.10). Once registration is completed, the accuracy is verified by ensuring that the predicted 1-mm error sphere completely encompasses the entire head and that the registration error is less than 0.7 mm (Fig. 21.2). More importantly, we assess the accuracy of the registration by touching several of the fiducials to verify that they are precisely located. Fig. 21.7 The reference arc is attached to the Nexframe base and fiducial are registered with the Nexprobe. Next, the dura is opened and any vessels in the path of the trajectory are coagulated and sharply divided. To prevent a cerebrospinal fluid leak and subsequent brain shift, which could reduce the accuracy during the procedure, the bur hole is sealed with a combination of Gelfoam and dural sealant. The alignment tower is then attached to the platform, aligned to the target through the neuronavigation system, and locked in placed. Next, the tower is secured to the platform (Fig. 21.11). The next stage, which employs the Ben-Gun (Schaerer-Mayfield, Lyon, France), entails the placement of one to five cannulas; we have found that accuracy is improved with cannulas that extend to 10 mm above the target in comparison to shorter cannulas. The rest of the procedure involves selecting the optimal track, by using microelectrode recording and macrostimulation, to assess for side effects and efficacy. The DBS lead is secured with the Stimloc and marked with a pen at the immediate junction of the DBS lead and the Stimloc. This mark provides visual confirmation that the lead has not moved during disassembly and is much more efficient than using repeated fluoroscopic images. Fig. 21.9 Placement of the Nexframe base. Screws are initially placed with a power screwdriver and then tightened by hand. Both the STarFix and Nexframe systems have the advantages of the frameless technology, but there are some differences; the main one is the method by which the trajectory alignment is done. The trajectory alignment of the STarFix system is based on traditional stereotactic frame principles and is set by the manufacturer, which can theoretically lead to error, but a group describing its recent experience with 263 patients has not encountered this problem.12 Furthermore, because the trajectory is set by the manufacturer, it does not allow for the flexibility to change the planned target. In contrast, the Nexframe system uses an optical tracking system for trajectory alignment during surgery, which allows for a change in the planned target. However, the system relies heavily on precise intraoperative registration and manual alignment of the trajectory. Another difference between the systems is that the STarFix system allows for simultaneous bilateral microelectrode recording, stimulation testing, and DBS placement, whereas the Nexframe system requires bilateral DBS placement to be done sequentially. Deep brain stimulation for Parkinson’s disease requires extreme accuracy, as it directly relates to efficacy.16–19 For example, the subthalamic nucleus (STN) is approximately 6 × 4 × 5 mm, and a DBS lead placement error of 3 to 4 mm can alter the outcome.26,27 The accuracy of stereotactic frames and frameless systems has been well studied in in vitro phantom experiments. The mean accuracy of the CRW and Leksell frames has been shown to be 1.7 ± 0.1 mm and 1.8 ± 0.11 mm, respectively.28 The mean accuracy for the Nexframe and STarFix systems has been measured to be 1.25 ± 0.6 mm and 0.42 ± 0.15 mm, respectively.21,29 Although the traditional frame-based stereotactic system has been used since the late 1940s, it was not until 2002 that the accuracy of the frame-based system in a clinical setting was established.30 Such clinical studies require a marker for actual target localization, with an internal (anterior commissure–posterior commissure [AC-PC]) or external (frame) reference system on the postoperative scan to compare expected with actual target locations. Alternatively, this process can be accomplished with image fusion, which is a relatively recent development. Starr and colleagues30 carefully assessed the postoperative coordinates of 76 STN DBS electrodes that had been placed with a Lek-sell frame. They found a mean deviation of 3.15 mm from the expected target location. Subsequently, similar results have also been reported by other groups.31,32 The University of California–Los Angeles group evaluated the discrepancy between expected and actual targets in 217 DBS cases. There was a mean vector error of 2.9 mm (range 0.1–6.44 mm) for the ventral intermediate nucleus, 2.3 mm (range 0–7.61 mm) for STN, and 2.2 mm (range 0.03–4.5 mm) for targets in the globus pallidus.31 O’Leary and colleagues32 analyzed intraoperative radiographic data on 109 micro-electrode tracks and found a 2.1-mm discrepancy between the theoretical microelectrode target and the values obtained from intraoperative fluoroscopic images. Schrader and associates27 assessed the electrode location in six patients who had the Zamorano-Dujovny stereotactic ring. The calculated vector error from their data was 2.64 mm for the left side and 3.04 mm for the right side. Hamid and coworkers33 examined the pre- and postoperative MRI scans to define the accuracy of lead placement in STN DBS for 16 patients who had the Leksell stereotactic frame; the calculated vector error was 3.02 mm. In 2005, the clinical accuracy of the Nexframe system was investigated by Holloway and associates.34 This multicenter study compared the accuracy of the Nexframe with the previously published data of frame-based clinical accuracy by Starr and colleagues.30 A total of 47 electrodes were implanted in 38 patients. The target nucleus was the ventral intermediate (VIM) in 16 patients, the STN in 29, and the globus pallidus pars interna (GPI) in two patients. Vector errors were analyzed in the same manner as in the frame-based study by Starr and colleagues by comparing the difference between the expected target and the actual lead location. Analysis revealed a vector error of 3.15 mm for the Nexframe, which was the same as the vector error of the frame as reported by Starr and colleagues. A follow-up publication from a single institution further investigated the clinical accuracy of the Nexframe and frame-based systems.35 Ninety patients underwent placement of 139 DBS leads with the CRW frame (n = 70) or the Nexframe system (n = 69). The final DBS location was identified on a postoperative CT fused to the preoperative CT and MRI scans. The vector error between the CRW frame (2.65 mm) and the frameless (2.78 mm) system did not differ (p = 0.69). The vector error for both systems declined with time, as the vector error of the last 20 implants was 1.99 mm for the CRW frame and 2.04 mm for the Nexframe. This decline was attributed to subtle changes in surgical techniques and experience. Another interesting finding from this study was the predictability of the vector error for the frameless system, with a proclivity toward the medial, posterior, and inferior directions, whereas the frame-based system did not have a predictable direction of error. The clinical accuracy of the STarFix system has also been examined recently by Konrad and colleagues.12 A total of 263 patients who underwent 284 DBS implantations with the STarFix system were analyzed. The final DBS lead location was calculated using postoperative CT scans. The mean vector error was found to be 1.99 mm (standard deviation: 0.9). Bjartmarz and Rehncrona36 directly compared the frameless and framed approaches in a single operation. In bilateral procedures during a single surgical session, one side was implanted with the Nexframe approach while the other side was implanted with a Leksell G frame. The preoperative planning image was based on a volumetric, 1-mm slice thickness, T1-weighted MRI study. Unlike the preceding studies, the DBS lead position was not assessed with a CT or MRI scan but rather with a two-dimensional X-ray image using the reference frame of the Leksell system. This method heavily favors the frame, as the ground truth is defined as the stereotactic coordinates in the Lek-sell space. With this method, the frame vector error was 1.2 ± 0.6 mm and the frameless error was 2.5 ± 1.4 mm (p < 0.05). It is unclear what the error would have been with the more commonly used method of postoperative imaging with CT or MRI studies, and there were other limitations to their frameless technique.37 Despite showing a difference in clinical accuracy between the frame and frameless techniques, however, the clinical outcome was similar at follow-up regardless of the stereotactic technique. Clinical studies show a greater error for both frame and frameless systems, as compared with phantom experiments. The increased error seen in the clinical situation is expected for several reasons, including weight bearing by the frame, mobility of the brain within the cranial cavity, loss of cerebrospinal fluid with subsequent brain shift, inaccuracies of localization introduced by selection of the lead tip and the AC-PC coordinates on postoperative imaging, and deviations of the microelectrode or DBS as it passes through the brain substance. Rohlfing and associates38 found a decrease in the accuracy of stereotactic frames because of torque introduced by the effect of weight bearing on the frame. They assessed the effects of the mechanical loading of the frame and a change in patient position on localization error within the clinical situation. They chose to compare scans obtained while the patient was prone and supine, maximizing the adverse effect of linear mechanical loading. CT scans were obtained in 14 patients placed in the Brown-Roberts-Wells frame while supine and then prone, and the registration transformations were compared. The mean error was 0.97 ± 0.38 mm, but the registration error was greater than 1.5 mm in eight of 14 patients. The authors noted that the errors from positioning and mechanical loading were additive with other sources of error. There are numerous pitfalls in attempting to measure postoperative lead locations accurately. First among these is the difficulty in locating the precise center and depth of the lead as it relates to the intended target. Both magnetic susceptibility artifact (on MRI) and beam-hardening artifact (on CT scans) conceal the lead and require estimation or interpolation of the electrode position. Papavassiliou and associates39 evaluated DBS lead locations in eight cases by using both CT and MRI studies. They found differences between the two techniques ranging from 2.4 to 2.6 mm; the mean of these signed values was 0.1 to 0.3 mm and was not significant. Furthermore, because the last contact of a Medtronic DBS lead lies 1.5 mm from the actual tip of the electrode, determining its location on either modality can be difficult. Because the DBS trajectory is not perpendicular to the AC–PC plane, an error in localizing the electrode tip can lead to errors not only in z (depth) but also in the x and y directions, depending on the approach angle. Even if the lead position can be accurately determined on postoperative imaging, relating this position to the intended target requires a translation method such as image fusion or coordinate transfer, each of which has potential inaccuracies. An additional category of error can relate directly to errors in lead placement. Some examples include deflection of the lead during implantation, slippage of the lead during anchoring, and various inaccuracies in stereotactic localization, which have been described elsewhere.28,29,38,40 The direct measurement of localization errors in phantom studies eliminates the step of translating postoperative imaging to preoperative targeting, which may contribute to the relatively decreased accuracy noted in clinical studies compared with phantom studies. In addition to studies that have focused on phantom and clinical accuracy of the frameless technique, two recent studies have compared the long-term clinical outcome between the frameless and frame-based techniques.41,42 The DBS Study Group analyzed the 1-year clinical outcome for patients with Parkinson’s undergoing STN DBS with the frameless technique.15 There were 31 patients, 28 with bilateral and three with unilateral lead placements. The Unified Parkinson’s Disease Rating Scale (UPDRS) was assessed at 6 and 12 months. All patients underwent DBS implantation with the Nexframe system. The mean improvement in UPDRS scores at 1 year was 58% with a mean reduction of medication of 50%. This level of clinical improvement is comparable to published frame-based clinical outcomes of 52 to 55%.43,44 In a similar study, Tai and colleagues42 directly compared the clinical outcome of patients with Parkinson’s undergoing STN DBS with frameless and frame-based techniques. A total of 24 patients were enrolled, 12 who underwent the frameless (Nexframe) technique, and 12 who had the frame-based (CRW) technique. At 1-year follow-up, the mean UPDRS improvement for the frameless (60.9%) and frame-based (56.9%) group was similar (p = 0.81). The frameless stereotactic system has been shown to be equivalent to the gold-standard frame-based system in experimental and clinical accuracy studies, as well as in clinical outcome studies. According to our experience and that of other published reports,12,23,34–36,41,42 several advantages related to the use of the frameless system have been noted. Patients are much less apprehensive about fiducial marker placement than the application of a stereotactic frame. The ability to apply the fiducial markers one or more days before surgery allows imaging and planning to be separated from the procedure, decreasing operating room time and enhancing the patient’s comfort because of the shorter periods spent without medication. Without rigid fixation to the operating table, patients are allowed greater mobility and seem better able to tolerate lengthy procedures. Intra-operative examination of the patient is easier without the bulky frame. As with any new device or procedure, however, the frameless system has a learning curve and requires attention to detail.35 We believe that the frameless stereo-tactic system is a safe, accurate, and effective technique and provides a viable alternative to the frame-based system. Parkinson’s disease (PD) is a progressive neurologic disorder, associated with the death of dopaminergic cells in the substantia nigra, but additionally with widespread effects across the entire central nervous system. Medical therapy for PD in the form of dopaminergic medications—most notably L-dopa—emerged in the 1960s45 and has shown unquestioned efficacy in reducing the severity of the cardinal motor symptoms of the disease. Unfortunately, a large proportion of PD patients become disabled within 5 to 10 years despite medical therapy because of the limitations of dopaminergic medications, including the predictable “wearing off” of drug effect over the course of a day, more unpredictable “on-off” motor fluctuations, and disabling involuntary movements now recognized as L-dopa–induced dyskinesias.46,47 In these patients, treatment with DBS may be a suitable option.48 Strong evidence now exists from randomized controlled trials supporting DBS as superior to best medical management for patients with moderate to severe PD.49–51 More than 80,000 DBS insertion procedures have been performed since the early 1990s, with an ongoing accrual of 8,000 to 10,000 patients annually.52 Bilateral placement of DBS electrodes into the STN has emerged as the most common procedure worldwide, and is typically done with frame-based stereotaxy and MRI-based target selection, complemented by intraoperative electrophysiological guidance with microelectrode recording. This section focuses on our frame-based approach to STN DBS, which we discuss in the context of the patient in this chapter’s case presentation. From the case description presented, a 65-year-old patient has been offered and has consented to STN DBS therapy. Before proceeding with the neurosurgical implantation of DBS electrodes, however, it is worthwhile to ask whether he is an appropriate candidate for this therapy altogether. Patient selection is critical to successful long-term outcomes in DBS; by some estimates up to 30% of DBS failures may be ascribed to inappropriate indications for surgery.53 At our center, the decision to offer DBS surgery to a given patient is based on a detailed and individualized analysis of several clinical factors. Central to this process is a multidisciplinary team composed of movement disorder neurologists, psychiatrists, and neuropsychologists, with the operating neurosurgeon being the ultimate arbiter. The ideal candidate who is likely to benefit substantially from STN DBS is one suffering from moderate to advanced PD complicated by motor fluctuations, disabling dyskinesias, or tremor despite optimized drug therapy.52,54 It is important to exclude patients with atypical parkinsonism or Parkinson plus syndromes such as progressive supranuclear palsy and multisystem atrophy, because these patients derive less benefit from DBS.55 Similarly, patients with a preponderance of axial disturbances such as postural instability or gait disturbance56,57 or prominent dysarthrophonia58 do not typically experience improvement in these symptoms with STN DBS. Additionally, the ideal candidate is also one whose cardinal motor symptoms of PD are responsive to L-dopa.44,59 At our center, all prospective patients undergo a formal L-dopa challenge, on which we require at least a 30% improvement in the UPDRS-III score. There is evidence that younger patients are more likely to do better with DBS,49,60,61 and although we do not have an absolute age cutoff, we are extremely cautious about offering surgery to patients older than 75 years. Moreover, advanced age correlates with the presence of dementia or cognitive impairment, both of which are exclusion criteria for STN DBS.62 As a rule, all patients in our practice undergo formal neuropsychological and cognitive testing before being considered for DBS. Finally, patients with unstable psychiatric conditions, particularly depression or impulsivity, must have their medication optimized before surgery because of the potential for deterioration in their psychiatric symptoms after STN DBS.61,63–65 We frequently consider alternate DBS targets—most commonly the internal segment of the globus pallidus—in patients with a significant psychiatric history who would otherwise be good surgical candidates. General medical fitness for surgery is also an important criterion.61 Conditions such as hypertension, coronary artery disease, and diabetes should be optimized medically before surgery, and the patient should understand that these conditions may increase their surgical risk. Antiplatelet or anticoagulant medications should be withdrawn in advance of surgery and will require special management in the perioperative period.66 Assuming the 65-year-old man in this case meets these stringent criteria, frame-based stereotactic surgery for DBS electrode implantation into the STN can be offered as a viable option and undertaken once consent is obtained. Several articles have described the various techniques used during the implantation of DBS systems.66–69 Neurosurgeons typically select their preferred techniques based on their training and experience and institutional resources.52 We highlight here some key points that we have used in our STN DBS procedures. We insert electrodes for STN DBS in conscious patients, with minimal or no sedation, in the off-medication state. In this way, we can maximize the quality of electrophysiological information obtained during intraoperative micro-electrode recording, and test the leads intraoperatively to determine their efficacy against PD symptoms and adverse effects, with the ultimate aim of optimizing electrode placement. In our view, frame-based stereotaxy is the standard for accurate delivery of the DBS electrode to the target. We place the head frame (Leksell G, Elekta, Atlanta, GA) on the morning of surgery with the patient seated on a chair. The frame is placed by the principal surgeon and an assistant, who maintains the base ring of the frame in line with the AC-PC plane, which is roughly approximated by the canthomeatal line.66 We liberally infiltrate the scalp at the pin sites with local anesthetic (1:1 mixture of 0.25% bupiva-caine and 1% lidocaine with epinephrine). We take care to carefully estimate the optimal pin length to prevent readjustments or replacements. Orthogonal pins are tightened simultaneously. Once the frame is in place, the patient is taken to the MRI scanner for preoperative imaging. We obtain a T1-weighted volumetric scan of the entire brain, as well as T2-weighted axial and coronal scans through the region of the STN. In total, the acquisition of all MRI sequences takes about 20 minutes. The patient is then taken to the operating room and prepared for surgery by the nursing and anesthesia team, while the neurosurgical team proceeds with anatomic planning on the recently acquired images. We use a combination of direct and indirect techniques to select the STN target on standard surgical planning software (FrameLink, Medtronic). Entry points and trajectories are also determined with navigation software. All target coordinates as well as frame arc and ring angles are verified independently by at least two different members of the neurosurgical team. In the operating room, the patient is placed in the supine position with the knees flexed and the head elevated so that the operating table takes on the shape of a reclining chair. Care is taken to ensure that the patient’s neck is in a comfortable position before affixing the head frame to the operating table. Draping is done is such a way as to allow access to the patient’s face, arms, and legs. The scalp is liberally infiltrated with local anesthetic near the coronal suture. We prefer a single Souttar transverse skin incision extending to either side of the midline for bilateral procedures. Standard techniques are used to drill the bur holes, open the dura, and insert the cannula for the microelectrode to an offset 10 mm above the target. Microelectrode recording is used in conjunction with clinical testing to ensure that the predetermined trajectory traverses at least a 5-mm segment of STN with kinesthetic-responsive units. Although the microelectrode recording data usually confirm the appropriate location of the MRI target within the motor territory of the STN, in roughly one of three patients the imaging target is suboptimal. Accordingly, an adjustment of 2 or 3 mm in the medial/lateral or anterior/posterior plane is usually required, although in some rare cases the error is greater. Because of this, we do not hesitate to record from additional trajectories as needed. Once a satisfactory final trajectory has been determined and any target modifications have been made, the DBS electrode is introduced into the cannula and advanced to the target under fluoroscopic guidance. Test macrostimulation through the DBS electrode is then done with an external screener, employing typical therapeutic stimulation parameters (e.g., 130 Hz frequency, 1–5 V amplitude, 90 µs pulse width). This should confirm beneficial effects for tremor, rigidity, and bradykinesia without adverse stimulation-related side effects, including motor phenomena (contralateral contractions or dysarthria), ipsilateral ocular adduction, or uncomfortable paresthesias, corresponding to electrode positions that are too lateral, medial, or posterior, respectively.66,70 Finally, the DBS electrode is fixed in place with a standard lead fixation system under fluoroscopy, and the entire procedure is repeated on the contralateral side. Postoperative MRI to verify correct electrode placement is standard practice at our center (Fig. 21.12), and we usually implant the pulse generator under general anesthesia a few days after the electrodes are inserted. The stereotactic frame has been a mainstay of functional neurosurgery since its development. Virtually all neurosurgeons who do modern DBS implantation procedures were trained to do so with strictly frame-based techniques, which have ultimately proven their worth with a stellar rec ord of safety and efficacy. Frameless stereotaxy has gradually become more popular in general neurosurgical practice over the past two decades,71 and is now the technique of choice in most centers for preoperative craniotomy planning, intraoperative neuronavigation, and to obtain stereotactic biopsies of intracranial mass lesions. It is only very recently, however, that frameless techniques have been used for functional work, in which the ultimate aim of correct electrode position places a premium on superb accuracy, which was long thought to be unattainable without a rigid head frame. Nevertheless, frameless approaches to DBS surgery are now increasingly in vogue be- cause of the widespread perception that they are minimally invasive.72
Deep Brain Stimulation: Frame vs. Frameless Stereotactic Treatment
Deep Brain Stimulation: The Frameless Technique
The Nexframe Stereotactic Technique
Phase 1: Preoperative Planning
Phase 2: Intraoperative Technique
Comparison of the STarFix and Nexframe Systems
Accuracy of the Frameless and Frame Stereotactic Systems
Conclusion
The Frame-Based Approach to Subthalamic Nucleus Deep Brain Stimulation
Case Analysis
Surgical Approach and Technique
Our Preference for the Frame-Based Approach
< div class='tao-gold-member'>
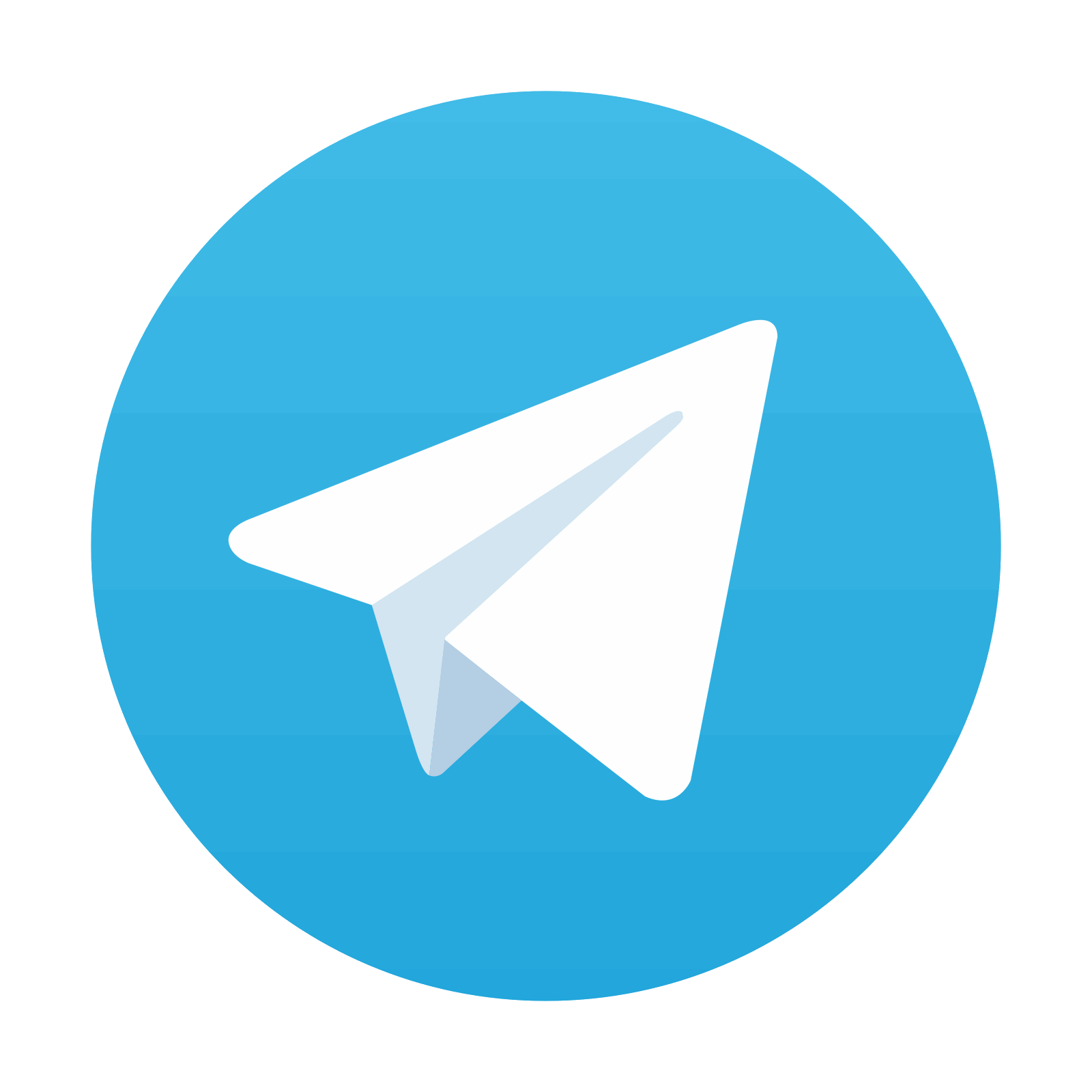