Chapter 11 Case A 35-year-old woman came to medical attention for a recent onset of seizures and headaches. Participants Glioma Resection in an Awake Patient with Cortical Stimulation: Mitchel S. Berger and Nader Sanai Optimal Resection of Insular Glima with Image-Guided Technologies: Sanju Lama, Stefan Wolfsberger, and Garnette R. Sutherland Glioma Resection in Eloquent Areas: Anatomical Basis and Resection with Tractography Studies: Uğur Türe and Pablo González-López Moderator: Insular Tumors: Allan Friedman The insular region remains one of the most challenging locations for aggressive resection of both low-grade and high-grade gliomas.1–12 These tumors are entrenched in eloquent tissue and surrounded by microvasculature that serves critical language and motor systems.13,14 Moreover, the insula itself is implicated in a variety of cognitive functions, including memory, drive, affect, gustation, and olfaction.15–17 Given the potential involvement (and impairment) of these essential neural networks, controversy persists as to which treatment strategy is appropriate for patients with insular gliomas and how intervention can have an impact on patient outcomes. Although observation, stereo-tactic biopsy, radiosurgery, and microsurgical resection have all been proposed,18–20 none has been studied in comprehensive detail in patients with insular gliomas. Insular gliomas are not rare, and, according to a recent epidemiological study by Duffau and Capelle,21 account for up to 25% of all low-grade gliomas and 10% of all high-grade gliomas. As we recognize the eloquence and complexity of the insular anatomy, we discern that insular gliomas can be distinguished from other gliomas in several respects. As sug gested by epidemiological data, there is a clear propensity for low-grade histology that is unique to the insula. Although the etiologic cause of this predisposition is not yet understood, this phenomenon may indicate a particular microenvironment within the insula or address the very nature of the glioma cells of origin propagating these tumors. Additionally, many patients harboring these tumors experience a prolonged and slowly progressive clinical course that is generally less aggressive than patients with similar lesions in other locations. Among tumors with both low- and high-grade histologies, this unpredictable natural history is characteristic of insular gliomas. Recent analysis, however, suggests that an insular glioma’s predilection for malignant transformation can be altered with aggressive cytoreduction.22 The patient’s history provides important information regarding the likely diagnosis of the tumor, and the neurologic examination can reveal the lesion’s location. Together with imaging, the history and physical examination are the foundation on which the perioperative assessment is established. Patients with insular gliomas often come to medical attention because of an ictal event (seizure), a change in functional capacity (paresis, vision changes, etc.), or headache and cognitive impairment (increased intracranial pressure). The speed with which these problems evolve is often proportional to the aggressiveness of the tumor (e.g., a rapid evolution of signs portends a rapidly growing lesion). However, slow-growing or low-grade lesions can remain clinically silent until they reach a critical size, at which time small changes in tumor volume can generate dramatic neurologic symptoms. Because the chronicity of the history gives some indication of the anticipated growth rate of the tumor, it is very important to inquire about signs and symptoms that may be considered unremarkable or unrelated by the patient. It is not uncommon, particularly in patients with low-grade insular gliomas, that symptoms precede identification of the tumor by many years. The most common undetected sign of a tumor is a partial seizure. Clues that suggest partial complex seizures include a history of problems in school or unexplained learning disabilities. A careful assessment of the patient’s academic history or work performance can uncover early evidence of a tumor that remained undetected. A change in function or capacity to perform at a consistent level may be misinterpreted as the result of stress or inattention when, in fact, the impairments may be the result of partial seizures. In addition, the rapidity with which signs and symptoms evolve gives an indication of how quickly intervention is needed. Progressive changes in functional capacity or altered consciousness are indications for more rapid intervention. Seizures are the most common sign of a tumor in patients with low-grade gliomas and are the first sign of a tumor in 80 to 90% of these patients. Because most of these tumors expand slowly, subtle changes in functional capacity are commonly overlooked or accommodated by the patient, and it is an ictal event that is the first indication of a structural problem. Progressive neurologic deficits occurring over a few days to weeks are more common in patients with high-grade gliomas. A more rapidly expanding mass lesion produces focal neurologic signs that bring the patient to medical attention. Once a diagnosis of insular glioma is suspected, surgical priorities should be directed toward establishing a diagnosis, relieving tumor mass effect, and maximizing cytoreduction. Surgical options for insular gliomas are limited to a biopsy versus resection. Stereotactic needle biopsy can obtain tissue for diagnostic purposes, but there is a risk of sampling error. In one recent study, overgrading of World Health Organization (WHO) grade II tumors occurred in 11% of cases and undergrading of WHO grade III gliomas in 28%.23 Thus, histopathological diagnosis of gliomas with only stereotactic biopsy comes with a substantial risk of inaccuracy, particularly for tumors with low proliferative activity or for mixed gliomas. At present, the indications for a biopsy in a patient with a presumed insular glioma are a diffuse lesion, such as gliomatosis, and the patient’s inability to undergo a definitive operation for medical reasons. Microsurgical corridors to the insula include transcortical and transsylvian routes.1,3,4,6,13,19 For select lesions, a transcortical “plus” operation allows the combined use of transcortical and transsylvian routes through a small split in the sylvian fissure. In a recent study comparing operative approaches to temporal mediobasal tumors, the transsylvian approach was associated with the highest combined rate of neurologic morbidity.19 A transsylvian route may be effective, but the risk of vascular injury and pial transgression during the course of exposure is not insignificant. To illustrate this point, a recent retrospective study of patients with insular gliomas treated at a single center noted that the transition from transsylvian to transcortical approaches was associated with a concomitant decline in neurologic morbidity.6 Nevertheless, the literature remains inconclusive for purely insular lesions, and therefore the use of a transcortical “windowing” technique or a transsylvian fissure split is a valid option. The tumor’s location and the patient’s hemispheric dominance determine the selection of either general or awake anesthesia. Our protocol for awake neuroanesthesia has been previously described.24 Patients are placed in a semi-lateral position with the head parallel to the floor. For tumors occupying the posterior aspect of the insula, turning the patient’s head 15 degrees upward allows for tumor resection underneath the lip of functional cortex overlying the posterior insula. The vertex of the head is tipped 15 degrees toward the floor or toward the ceiling when the tumor is predominantly below or above the sylvian fissure, respectively (Fig. 11.1). After a tailored craniotomy, cortical and subcortical language are assessed and motor and sensory mapping are conducted as needed and according to previously reported protocols.24 Once functional areas have been identified, transcortical windows above and below the sylvian fissure are created through nonfunctional cortex, taking care to maintain at least a 1-cm margin from any functional language site. For motor sites, however, the 1-cm rule need not apply. This “windowing” technique allows for tumor resection along the course of the uncinate fasciculus, generating suprasylvian and infrasylvian resection cavities that are eventually connected to one another underneath the skeletonized sylvian vessels (Fig. 11.1). Finally, identification of the lenticulostriate arteries25 and motor mapping of the internal capsule 26 allow for delineation of the medial border of resection in most cases. For each patient, the insula can be divided into four zones and the tumor’s location can be assigned to one or more of these zones (Fig. 11.2). Along the horizontal plane in a sagittal view, the insula is first bisected along the sylvian fissure. A perpendicular plane is then intersected with this at the level of the foramen of Monro. The resultant quadrants (anterior-superior, posterior-superior, posterior-inferior, and anterior-inferior) are designated zones I, II, III, and IV, respectively (Fig. 11.3). Tumors that occupy more than one zone are noted as such (e.g., zone I + II). When the tumor occupies all four zones, these insular gliomas are defined as “giant.” The anterior zones of the insula most frequently harbor insular gliomas. An analysis of the extent of resection, however, confirms that these zones are also most amenable to a greater extent of resection.22 In contrast, more posterior lesions, such as those of zone II (posterior-superior), are associated with a lower mean extent of resection, likely because of their close proximity to the rolandic cortex, inferior parietal language sites, and the posterior limb of the internal capsule. Nevertheless, with the appropriate use of cortical and subcortical motor and language mapping techniques, a mean extent of resection of 67.4% can be achieved for zone III insular gliomas and a greater than 95% extent of resection for tumors in zone I.22 Overall, it is feasible to aggressively resect gliomas of all grades in the insula, and the morbidity profiles reported in recent years indicate that this can be done relatively safely and without the neurologic morbidity that detracts from the quality of life.6,9,22,27 Fig. 11.1a–e The patient’s position and microsurgical technique for transcortical resection of insular gliomas. For tumors primarily below the sylvian fissure, the head is tilted 15 degrees below horizontal (a), while it is tilted 15 degrees above horizontal (b) for tumors primarily above the sylvian fissure. After cortical mapping identifies areas of nonfunctional cortex, a series of cortical windows (c) is made above and below the sylvian fissure. (d) Tumor resection traces the course of the uncinate fasciculus, eventually connecting the suprasylvian and infrasylvian windows. (e) Lenticulostriate arteries are identified at the medial margin of resection, along which the internal capsule can be subcortically stimulated. MCA, middle cerebral artery. (From Sanai N, Polley M-Y, Berger MS: Insular glioma resection: Assessment of patient morbidity, survival, and tumor progression. J Neurosurg 112(1): 1–9, 2010. Reprinted by permission.) The heterogeneous nature of gliomas is evidenced by the diversity in histology, genetics, and locations exhibited by these tumors. Our experience with insular gliomas suggests that this particular subtype behaves differently from tumors of a similar grade located elsewhere. Specifically, gliomas located in the insula are associated with a prolonged clinical course characterized by comparatively longer intervals of overall survival and progression-free survival. In recent studies of grade II and III gliomas in all locations, the median times to progression were 5.5 years28 and 2.1 years,29 respectively. Comparatively, patients with grade II and grade III insular gliomas described in recent work have had longer progression-free survivals.22 The magnitude of difference, however, remains unclear, as most published studies have an insufficient duration of clinical follow-up. Nevertheless, such a unique natural history applies to grades II, III, and IV insular gliomas, raising the possibility that the insular microenvironment, a shared histopathological trait, or a combination of the two may be driving this clinical behavior. Aggressive resection, however, cannot be advocated without evidence of an improved outcome. This remains one of the most challenging dilemmas in neurosurgical oncology, as much controversy surrounds the value of a greater extent of resection for gliomas.30 In some studies, however, focusing the analysis on a homogeneous subset of gliomas reveals the unambiguous value of greater resection.31 Whether this indicates the heterogeneity of gliomas or that certain subtypes are more amenable to resection remains unknown. Nevertheless, among purely insular gliomas, a statistical correlation between greater extent of resection and critical patient outcome parameters, including overall survival, progression-free survival, and malignant progression-free survival, has been shown.22 This relationship exists among both low-grade and high-grade gliomas, consistent with the hypothesis that insular gliomas, irrespective of grade, follow an indolent clinical course that can be positively affected by aggressive resection. We recently reported that the interval to malignant progression among grade II insular gliomas is longer for patients who have had greater resection.22 The biological basis for this alteration remains unclear, but may be related to the volume of residual tumor as a predictor of malignant transformation.29 Given the dramatic differences in clinical behavior and outcome between grade II and III gliomas, this represents a potential shift in our concept of aggressive glioma resection. The ability to manipulate the natural history of these tumors makes a case for earlier intervention in the microsurgical treatment of insular gliomas and argues against the validity of a simple biopsy or a “wait-andsee” approach. Fig. 11.2a–c The Berger-Sanai insular glioma classification system. (a) Zones I to IV are divided along the line of the sylvian fissure and a perpendicular plane crossing the foramen of Monro. (b) The tumor’s location is determined according to the location of the majority of the tumor mass. (c) Axial illustrations of zones I and IV, located anterior to the foramen of Monro, and zones II and III, located behind the foramen of Monro. (From Sanai N, Polley M-Y, Berger MS: Insular glioma resection: Assessment of patient morbidity, survival, and tumor progression. J Neurosurg 112(1): 1–9, 2010. Reprinted by permission.) For the neurosurgeon, insular gliomas represent an opportunity to intervene and have an impact on outcome in several ways. Aggressive resection of insular gliomas of all grades can be accomplished with an acceptable morbidity profile and is predictive of an improved overall survival and progression-free survival. The extent of resection has been increasingly shown to correlate with an improved outcome, as well as with better seizure control and reduced malignant transformation rates. Neurologic morbidity can be minimized by combining traditional transcortical or transsylvian neurosurgical approaches with intraoperative stimulation mapping to identify functional and nonfunctional sites in and around the insula. Fig. 11.3 Preoperative T2-weighted axial and T1-weighted sagittal magnetic resonance imaging (MRI) for zones I to IV of the Berger-Sanai insular glioma classification system. Giant tumors are those that occupy all four zones. (From Sanai N, Polley M-Y, Berger MS: Insular glioma resection: Assessment of patient morbidity, survival, and tumor progression. J Neurosurg 112(1): 1–9, 2010. Reprinted by permission.) The management of insular gliomas remains controversial primarily because of the relationship of the tumor to adjacent vessels and eloquent brain, resulting in various therapeutic options including observation, biopsy, subtotal resection, and resection with a volume reduction of greater than 90%. Observation has been recommended as insular gliomas have been found to generally follow a slow and protracted course. Because of the heterogeneous nature of gliomas, conventional magnetic resonance imaging (MRI) with contrast enhancement may underscore its true grade. Up to 10% of nonenhancing low-grade gliomas are subsequently classified as high grade.32,33 Although subtotal resection is accompanied by a decreased operative risk, it may not have an impact on the patient’s outcome or the tumor’s progression to a higher grade. There is a growing consensus that the extent of resection correlates with an improved outcome for patients with both low- and high-grade tumors and that gross total resection of a low-grade glioma decreases the probability of its malignant transformation.22,28,30,34,35 Several groups have shown that low-grade insular and hemispheric gliomas can be safely approached, with a volumetric surgical resection of greater than 90% often achieved. Furthermore, investigators have suggested that the extent of resection of insular gliomas, irrespective of the grade, correlates with an improved outcome. However, because of the absence of randomized controlled clinical trials, establishing a definitive guideline for operative management remains controversial. Nevertheless, surgical excision is currently the preferred treatment option. Given the current evidence, maximal cytoreduction with the preservation of neurologic function can be considered the goal of modern glioma surgery. Over the past decades, several surgical advances have made safe resection of these complex tumors feasible. These advances include pharmacological brain decompression, physiological monitoring of the speech cortex and motor tracts, awake craniotomy, improved instrumentation, surgical planning with advanced MRI sequences including functional magnetic resonance imaging (fMRI) and diffusion tensor imaging (DTI), surgical navigation based on optical or electromagnetic tracking, intraoperative magnetic resonance imaging (iMRI) to correct for brain shift, and, more recently, image-guided robotic technology for improved precision and accuracy. Magnetic resonance imaging technology has been transported into the operating room to improve the intraoperative localization of lesions and control resection.36–39 These systems were initially of an open configuration and contained relatively low field magnets. Signal-to-noise and contrast-to-noise ratios have improved with the evolution of iMRI systems to higher field magnets, which are not open. These closed systems interrupt surgery for imaging and are therefore generally used to evaluate the extent of surgery rather than to guide it. Despite this challenge, unsuspected residual tumor has been reported in up to 50% of cases in which MRI was used during dissection.40 In a recent randomized clinical trial, the extent of resection and outcome were better in patients with gliomas treated with iMRI compared with conventional microsurgery.41 Furthermore, iMRI together with multimodal neurophysiological monitoring have been shown to allow an extended and safe resection of gliomas near eloquent cortex.42 In another study, among 89 patients with insular gliomas randomized to either microsurgery with iMRI or microsurgery alone, the extent of resection was greater in the iMRI-with-microsurgery group compared with the microsurgery-only group.43 These studies indicate that the extent of resection is greater when using iMRI. In an effort to increase the precision and accuracy of surgery, robotic technologies are being developed and merged with microsurgery,44–48 and recent advances have initiated remote surgery with sensory immersive capabilities.49,50 Posted at a workstation, the surgeon is able to take full advantage of imaging data while carrying out microsurgical dissection. The patient presented in this chapter is a 35-year-old woman with a recent onset of seizures and headache. It is assumed that her neurologic examination is unremarkable and that she is being treated with antiseizure medication. MRI studies show a large left nonenhancing cystic insular lesion consistent with a glioma (see images in the case description). The lesion spares the basal ganglia. The absence of significant contrast enhancement favors the diagnosis of a low-grade lesion. It is recommended that the patient proceed to open surgery for definitive tissue diagnosis and gross total resection of the lesion. For surgical planning, further MRI studies are advised. The iMRI and image-guided robotic technology can be used to maximize safety and the extent of resection. For this patient, the goals of surgery are as follows: • To obtain a definitive histopathological diagnosis for treatment planning • To achieve maximal surgical resection of the tumor while preserving language and motor functions, which will improve the outcome, delay progression, and delay malignant transformation • To potentially alleviate seizures and headache Gliomas of the insular region pose a considerable surgical challenge relating to neural connectivity and vascular structures critical to language and motor function. Precise anatomic knowledge of the insula forms the foundation of surgical planning. The insula is a well-defined anatomic structure buried deep within the sylvian fissure, covered by frontal, parietal, and temporal opercula.51 It is triangular in shape, with the margin formed by the circular sulcus that is anatomically divided into anterior, superior, and inferior limiting sulci. There are two surfaces of the insula—anterior and lateral. The fronto-orbital operculum covers its anterior surface, whereas the lateral surface is covered by the frontoparietal operculum superiorly and the temporal operculum inferiorly. Phylogenetically, the surface of the insula consists of mesocortex situated between the allocortex medially and the isocortex laterally. The allocortex consists of the amygdala and hippocampus, whereas the isocortex comprises the neocortex.51 The vascular supply originates from 8 to 12 branches of the M2 segment of the middle cerebral artery.52 The limen insulae, where the insular cortex is in continuity with the frontal operculum, is supplied by distal M1 branches and occasionally from the middle cerebral artery bifurcation. The insular arterioles supply the cortical and subcortical areas of the insular gyri and extreme capsule but not the external capsule, putamen, globus pallidus, or the internal capsule. This information is important, and with this knowledge the surgeon can perform coagulation with division of these arteries for tumor removal. The lenticulostriate vessels may be markedly displaced but they provide an important anatomic landmark during surgery, and they must be preserved.26,53 The normal insular cortex has been recognized for interoceptive awareness, that is, sensing the physiological condition of the body.54 Functional MRI in humans has shown the insula and anterior cingulate cortex to be the critical and sole substrates for such awareness, and therefore to be important in representing the visceral state of the body.55 Apart from language functions and sensorimotor integration, this region also accounts for cognitive and emotional processing; gustatory, auditory, and vestibular functions; and neuropsychiatric disturbances. Although data about the connectivity of the insula is sparse, DTI studies have shown important structural and functional connectivity.56 The ventral-most anterior insula is functionally interconnected to the rostral anterior cingulate cortex, the middle and inferior frontal cortex, and the temporoparietal cortex. The dorsal posterior insula is connected to several cortical regions, such as the dorsal posterior cingulate, premotor, supplementary motor, temporal, and occipital cortical areas. Thalamic projections to and from the insula remain less clear in human studies, unlike in other species in whom complex, region-specific thalamic projections to both anterior and posterior insula have been described.57 Given the importance of the insula and its anatomic and functional relationship to the surrounding structures, it is necessary to obtain detailed preoperative clinical and imaging data on the patient before planning surgery. This information will help not only in planning the surgical resection of the lesion but also in anticipating potential complications for an optimal resection and outcome. With the appropriate use of technologies, surgical intervention can provide a definitive diagnosis of both the tumor type and grade and will decrease this patient’s almost certainly elevated intracranial pressure, ultimately improving outcome. As part of the strategy for gross total tumor resection, diagnostic MRI studies are complemented with fMRI to delineate the speech and motor cortex.58,59 From these regions of interest, a 3D DTI tractography is reconstructed to visualize the relationship of important fiber tracts that relate to the lesion6: the arcuate fasciculus, which is located in proximity to the superior limiting insular sulcus, and the cortico-spinal tract within the posterior limb of the internal capsule. By fusing the data available from these complementary imaging studies, the surgeon can outline the preoperative planning of the approach and the extent of resection. It is probable that this chapter’s patient has some bilateral representation for speech, given the location of the tumor and her sex.60,61 Although not anticipated, fiber tracts within the neoplasm would limit tumor resection.62 Integration of the intraoperative imaging studies with the NeuroArm navigation system (IMRIS, Inc., Minneapolis, MN) further validates the accurate registration of the lesion’s location and the associated anatomy of functional, vascular, and fiber tracts that are instrumental for safe resection. Brain shift during surgery invalidates the navigation technology used for preoperative images.63–65 Although three-dimensional reconstruction of fiber tracts provides an excellent pictorial representation of the otherwise not visible fiber pathways and direction within the white matter, there remains a variable degree of uncertainty as to a real-time verification of such pathways. In other words, are the fiber tracts in real time where they appear to be on images? If so, what is the margin of error for a fiber tract representation in DTI and real time? Authors of cortical and subcortical stimulation studies, using a current intensity ranging from 2 to 8 mA, have reported the safe detection of fiber tracts at approximately a 5-mm distance from the site of stimulation.66 In particular, investigators have shown the precise and alternate resection of thin tumoral layers with electrophysiological stimulation to detect pathways such as the pyramidal tract posteromedial to or the arcuate fasciculus superomedial to the lesion.66,67 It has also been shown that the medial border of the resection is defined by the presence of the lenticulostriate arteries and functionally by the detection of the internal capsule and the pyramidal pathways. Originating as histopathological low-grade tumors, gliomas have an inherent tendency to progress to malignant high-grade tumors over time. This progression originates in a localized tumor area because of focal genetic changes and causes the well-known tissue heterogeneity of gliomas.68 Therefore, tissue sampling during open surgery may miss such an “anaplastic focus” in a large, otherwise low-grade tumor and delay the implementation of postoperative radiation and chemotherapy. Identifying an anaplastic focus within a low-grade glioma is mandatory for tissue sampling. To accomplish this task, the following visualization modalities have been suggested: • Magnetic resonance tomography shows areas of significant contrast-enhancement on T1-weighted MRI to indicate focal malignant progression and is routinely used to target tissue sampling. Most insular gliomas are non-enhancing; however, they may show the foci of a higher grade tumor. • Magnetic resonance spectroscopy enables the intratumoral detection of the most malignant areas through the display of metabolite distribution. Proton magnetic resonance spectroscopy has been found especially useful for diffusely infiltrating gliomas with nonsignificant contrast enhancement.69 The typical metabolite profile of glioma tissue includes an increased choline peak (resulting from elevated turnover of the cell membrane), a decrease in N-acetylaspartate (because of impaired neuronal function), and a reduction in creatine (stemming from the hypermetabolic state).70 Multivoxel spectroscopy, termed chemical shift imaging, has been successfully used for navigation-guided tissue sampling of the most malignant areas of gliomas. • Positron emission tomography that uses amino-acid tracers such as 42C-methionine or 18F-fluoroethyl-Ltyrosine is an established modality for imaging malignant foci in diffusely infiltrating gliomas. These images can be used to define the target for neuronavigation systems that guide tissue sampling.33 • Five-aminolevulinic acid (5-ALA), a precursor of the porphyrin synthesis pathway, has been found to accumulate in malignant glioma cells as strongly fluorescing protoporphyrin IX.33 Generally, if open neurosurgical resection of a low-grade glioma is attempted, the progressive brain shift usually renders navigation inaccurate and, consequently, precise tissue sampling becomes impossible. Incorporating 5-ALA into the procedure provides a more reliable intraoperative technique for visualizing anaplastic foci independent of brain shift. This compound can be excited with a violet-blue light source at 440 nm, and the resulting fluorescence at 635 nm then displays the anaplastic focus in an otherwise low-grade glioma. A recent study suggests that 5-ALA is a promising intraoperative marker for anaplastic foci in diffusely infiltrating gliomas with nonsignificant contrast enhancement on MRI independent from brain shift. We recommend that the surgeon take full advantage of this variety of imaging modalities as well as other technologies that will benefit neurosurgical planning and intervention. A high-field movable 3.0 T iMRI system, robot-assisted neuronavigation, and image-guided microsurgery with electrophysiological monitoring present a safe multimodal method for the surgical treatment of insular lesions. This approach provides both a mechanism for maximum control of resection and a more comprehensive framework for preserving the patient’s neurologic function and quality of life. After the patient is positioned and anesthesia is induced, images are acquired for surgical planning at the iMRI suite (Fig. 11.4). These images—T1-weighted with contrast enhancement, T2-weighted, and DTI—are used for robot neuronavigation. Fusing the images facilitates the concurrent display of anatomic and functional information (with DTI tractography and fMRI) to set electronic tumor boundaries and no-go zones (Fig. 11.5) together with metabolic imaging data to assess and target an anaplastic focus. A left frontotemporal craniotomy is performed in the usual fashion. The dura is opened in a cruciate manner to expose the sylvian fissure and the adjacent frontal and temporal opercula. The left sylvian cistern is opened to allow brain decompression. At this stage of the procedure, the robot is brought to the surgical site and re-registration is done (Fig. 11.6). Robotic dissection complemented with an assisting surgeon begins within the left sylvian cistern, and the M1 and M2 segments of the middle cerebral artery are defined together with the lenticulostriate and insular branches. Branches to the insula arising from the M2 segment are coagulated and divided. The tumor is entered at the predefined target point and initial biopsy specimens are obtained from the pre-registered areas of highest metabolic activity and sent to the lab for histopathology analysis, including frozen section. The tumor is debulked starting from the central aspect of the neoplasm with the aid of the robotic suction tool (Fig. 11.7). At progressive stages of the procedure, the robot and microscope are removed from the operative site and the magnet is returned for interdissection T1-weighted, T2-weighted fluid-attenuated inversion recovery (FLAIR), and DTI magnetic resonance imaging studies. Once these data are obtained, the magnet is removed from the operating room and re-registration to the updated images is done. Dissection continues out toward the tumor–brain interface defined on the MRI studies. As dissection approaches the corticospinal tract visualized on DTI tractography, the margin of safety is assessed with subcortical electrical stimulation. Resection is limited upon attainment of motor stimulation with 5 to 7 mA.71,72 Fig. 11.4 Surgical planning for intraoperative MRI, showing the magnet moved into position over the patient. The surgical treatment of gliomas in eloquent cortex has remained controversial, with recent meta-analysis data supporting extensive resection.30 The anatomic location of the lesion, however, poses a significant challenge in preserving function, specifically in relation to speech and motor power. After a thorough preoperative workup, a representative tissue biopsy of the lesion and the intent to carry out maximum resection are currently considered the goals of modern glioma treatment. The use of important surgical adjuncts—intraoperative electrophysiological monitoring and precise and accurate microsurgical techniques such as MRI-compatible surgical robots—maximizes resection and functional outcome concurrently. Such technology incorporates a high level of finesse and dexterity into the surgical procedure, which is essential for the optimal management of insular gliomas. Interdissection MRI enables a near real-time assessment of brain shift as resection progresses. In a patient with an insular glioma, this is especially important because the surgeon relies on MRI-based cues to resect residual tumor, as visual and haptic feedback is somewhat limited within the white matter. As the surgeon approaches the tumor margin, intraoperative electrophysiological monitoring adds to the safety of the resection by providing important information about the integrity of white matter tracts as well as the proximity of these tracts to the surgical dissection. The workstation allows the surgeon to take full advantage of imaging data during surgery. The workstation is completed by a robotic system that allows the surgeon to remotely perform the operation and effectively communicate with the surgical team. A combination of the improved precision and accuracy of the machines together with the dexterity and speed provided by the assistant surgeon optimizes the performance of surgery. In a retrospective review of 104 patients with a low-grade glioma of the insular region, the extent of resection correlated with a progression-free interval.22 This interval was defined as the time between initial surgery and the demonstration of an unequivocal increase in tumor on follow-up imaging, malignant progression, and/or death. In patients who underwent extensive resection (> 90%), malignant transformation of the tumor (selected for patients with grade II gliomas) was delayed, as shown by radiological enhancement on follow-up imaging studies, histopathological diagnosis of a higher grade on subsequent surgery, or both. Furthermore, extensive tumor resection has been shown to prolong the median time to disease progression.28,29 It is important, however, to reiterate the notion that a lack of enhancement on MRI studies does not establish a definitive diagnosis of a low-grade lesion. The existing literature shows no definitive consensus on whether the extent of resection improves the patient’s survival and quality of life. Current evidence from insular glioma surgery, however, provides mounting evidence for maximum resection.22,30,66 Seizures are one of the most common presentations of low-grade gliomas, and intractable seizures are observed in 10 to 20% of patients.73–75 In insular gliomas, which make up approximately 15% of low-grade gliomas, seizures are a predominant presenting feature and greatly impair the patient’s quality of life. One of the main indications for surgical removal of this lesion is therefore to render the patient free of seizures. The mechanism behind seizure activity and treatment strategies remains largely unknown.76,77 Despite the technical challenge pertinent to the insula and the variable tumor grade, surgical removal has been shown to benefit this cohort of patients. In this context, we believe that removing an insular glioma should alleviate a patient’s seizures. The technique of language mapping historically evolved from epilepsy surgery, in which a large exposure allowed extensive mapping of multiple cortical regions for language and motor functions before surgery as well as intraoperatively through awake craniotomy.78,79 A similar technique is widely used in surgeries related to lesions of the dominant hemisphere. Brain tumors have considerable topographic variability because of mass effect and functional reorganization that takes place over time through inherent plasticity mechanisms.66,80 Furthermore, individual variation among patients in their language representation, whether lateralized or bilateral, cannot be emphasized too greatly. Therefore, the surgeon must take into account the various fMRI evidence of language function areas near the tumor tissue while planning the surgical resection. Irrespective of tumor type, the use of intraoperative cortical and subcortical stimulation to accurately detect functional regions and pathways remains a key factor in the safe removal of insular gliomas of the dominant hemisphere. Although an awake craniotomy allows the surgeon to assess and preserve these functions, a combination of image guidance, iMRI, and electrophysiological monitoring is preferred in the anesthetized patient. Surgical planning based on multimodal imaging, together with a fusion of technologies for precise and accurate excision of the target, can help the surgeon achieve the therapeutic goal of gross total resection. Although the possibility of functional brain tissue within the lesion remains a matter of debate,62 continuous electrophysiological stimulation can potentially alleviate the risks of unwanted injury. Earlier in the disease progression, insular lesions can be typically infiltrative in nature. As the disease progresses, however, it tends to displace structures, thus allowing more room for central debulking.81,82 A considerable number of patients have new neurologic deficits after an attempt at maximum surgical resection of insular gliomas.30,83 This has been observed despite the use of electrophysiological monitoring, and is in part because of maximizing the resection to the functional boundaries intraoperatively. If such boundaries are respected with the application of modern surgical technology, such deficits can be expected to be transient and reversible. It is therefore important to include these variables in a neurologic rehabilitation management plan. From what is currently known about insular gliomas and their surgical management, the indication for surgery for tissue diagnosis is apparent. The extent of resection for maximum benefit in terms of clinical outcome, such as tumor progression, malignant transformation, the propensity for seizures, and overall survival, remains a matter of debate and discussion. The general incidence of insular gliomas is relatively low, but the technical and surgical challenges of carrying out such resections are profound. Although the evidence appears to support the surgical resection of such a lesion, the complex nature of the disease warrants referral to neurosurgical centers of excellence for the best outcome. The available imaging studies for the patient presented in this chapter indicate a nonenhancing intrinsic mass located in the anterior aspect of the left insula, showing paramagnetic features of hypointensity on the T1-weighted MRI sequence and hyperintensity on the T2-weighted sequence. The lesion spares the adjacent temporal, fronto-orbital, and frontoparietal opercula, and has a clear border with the putamen, which is pushed medially and posteriorly. The internal capsule, primarily the anterior limb, is also moved posteromedially. The clinical and radiological findings strongly suggest the diagnosis of a left insular, possibly low-grade, glioma. Brain tumors in eloquent areas present a great challenge for neurosurgeons because of the difficulty in removing them without causing neurologic disturbances. The integral management of these lesions entails using a variety of elements in an optimal perioperative approach: diagnostic and preoperative functional techniques, a clear understanding of neuroanatomy, intraoperative monitoring, and appropriate microsurgical technique. These elements all contribute to increasing the surgeon’s experience and anatomic knowledge, which are the only constant tools. In our experience, and supporting Yaşargil’s findings, gliomas do not originate from highly functional areas such as the motor or primary visual cortex (Brodmann areas 4 and 17). These tumors mainly affect eloquent regions by directly compressing the surrounding areas. Complete surgical resection seems to be the best treatment for low-grade glial tumors.29,30,84–86 Until new treatments are developed, the cytoreduction provided by surgery will be of value because it collects material for diagnosis and research, alleviates both neurologic and irritating impairments (as well as symptoms of intracranial hypertension), and increases the time to malignant progression.22,30 Following this line of reasoning, evidence supports the idea of resecting the largest possible volume of tumor.30,87–89 But the price to pay for such radical resection may be an increase in morbidity, a fact that is most important when eloquent areas are involved. To combat this problem, a great number of imaging, neurophysiological, neurochemical, and surgical techniques have been recently developed and incorporated into the general management of these tumors, with the ultimate objective of optimizing the resection and extending it to the maximum while minimizing the eventual associated morbidity.90–92 Nevertheless, the diagnostic and therapeutic armamentarium for gliomas should never obscure or replace the surgeon’s strong knowledge of neuroanatomy. In the human body, especially in the brain, pathology follows the anatomy, and neurosurgeons should therefore accurately study the different cerebral functional compartments. After observing and treating thousands of gliomas, Yaşargil and his colleagues introduced the concept of functional units. He argued that gliomas tend to initially develop in a single functional unit, pushing away the surrounding compartments according to the location where they grow. This general behavior seems to apply to almost all low-grade lesions and to high-grade gliomas during their initial stages. However, recurrent, irradiated, and high-grade lesions in advanced stages may transgress the borders of those functional compartments, a fact that reinforces the importance of a total resection during the first procedure. In general, the term eloquent area in the human brain refers to those regions with clearly demonstrated high functionality, where surgical or pathological disruption will unfailingly lead to neurologic impairment. This definition of eloquence is misleading, however. In this sense, many territories that are not considered eloquent may require surgical corridors related to highly functional compartments, as well as neighboring vascular structures feeding distant eloquent regions. In this perspective, eloquent regions should include motor, speech, and visual cortical areas with their white matter pathways, such as the pyramidal tract, superior longitudinal fasciculus (SLF), and optic radiation, and also the central nuclei such as the thalamus, hypothalamus, and basal ganglia, and certain regions in the limbic and paralimbic territories, brain stem, and deep cerebellar nuclei and peduncles. In short, with respect to function, neighboring anatomy, and surgical approach, most central nervous system lesions can be considered related to eloquent areas.93 The insular region perfectly illustrates this concept. As it would be too extensive to describe the general surgical management of tumors in the so-called eloquent areas, we consider the surgery of insular tumors as the perfect paradigm to illustrate this topic. In about the fifth month of embryological development, the cortical shield covering the basal ganglia and outlining the internal capsule forms the insula. Later on, this primitive insula begins enfolding in a central position because of the disproportionate growth of the adjacent neocortical areas, and is finally covered by the frontal, parietal, and temporal opercula. The insula of Reil belongs to the paralimbic system and represents a transitional element between the allocortex and the neocortex of the human brain. The paralimbic areas are commonly termed mesocortical because of their intermediate cortical architectonic features, and include the orbitofrontal cortex, insula, temporal pole, and parahippocampal and cingulate gyri. The gradual cortical differentiation between the old, and centrally positioned, limbic brain and the new neocortical areas takes the insula as its anatomic and cytoarchitectural link.94 The insula has connections to and from a large number of different regions within the human brain, and several higher functions have been attributed to this hidden structure, such as temperature, touch, fear, conscious desires, olfactory and gustatory inputs, subjective emotional experiences, the perception of effort during exercise, the regulation of higher cognitive functioning, and the processing of pain, as well as somatic functions, autonomic regulation, verbal working memory, and secondary motor and language control.95–99 Since the first insulectomies were done, however, some controversy has surrounded this area as a clearly defined functional entity. Surgery in this region may induce postoperative hemiparesis as well as different degrees of speech impairment when dealing with the dominant hemisphere. Mutism and apraxia after damage to the nondominant insula have also been reported. In most of these cases, however, postoperative pathological examination revealed damage to the surrounding opercular cortex and corona radiata, probably resulting from opercular and subcortical involvement of the lesion or direct surgical manipulation of these neural structures and ischemic injury from manipulation of the branches of the middle cerebral artery (MCA).98,100–102 The insula remains hidden in the depths of the sylvian fissure and forms the medial aspect of the sylvian fossa. To approach it, the surgeon should open the sylvian fissure and interopercular sulci. The sylvian fissure is divided into two compartments. The anterior or sylvian stem runs from the bifurcation of the internal carotid artery just inferior to the sylvian vallecula and, following the path of the sphenoid wing, reaches the level of the pars triangularis at the frontoparietal operculum. It courses between the orbital gyri and the polar planum in the anterior aspect of the medial surface of the temporal operculum. The place where the anterior stem becomes the posterior or insulo-opercular compartment at the level of the pars triangularis is named the sylvian point, and is an important landmark when splitting the sylvian fissure. The insulo-opercular section is divided into three main rami with a common origin at the level of the sylvian point. The horizontal ramus runs forward between the pars orbitalis and pars triangularis, whereas the ascending ramus runs upward, separating the pars triangularis and pars opercularis. The posterior ramus runs backward in a moderate undulating path toward the supramarginal gyrus, where it opens into ascendant and descendant terminal limbs.17 The posterior ramus is the longest of the fissure, and represents the cortical translation of the anteroposterior extension of the insula. It runs from the region of the pterion to its termination in the inferior parietal lobe, separating the frontoparietal and temporal opercula and forming the sylvian line. Its course seems partially broken by some subsulci that enter the frontoparietal and temporal opercula. The most constant subsulci are the diagonal sulcus in the pars opercularis, the anterior and posterior subcentral sulci delimiting the subcentral gyrus, and the side branch of the transverse temporal sulcus17 (Fig. 11.8). Fig. 11.8a–c Lateral view of the left hemisphere of a cadaveric specimen in which the main anatomic landmarks surrounding the sylvian fissure are marked. (a) The fronto-orbital, frontoparietal, and temporal opercula cover the insula. (b) The frontoparietal operculum has been removed, and the superior surface of the hidden insula is shown in the depth of the sylvian fissure. (c) A closer view in which the temporal operculum is being retracted downward to expose the entire surface of the insula. This maneuver provides a better view of the inferior surface of the insula to the level of the inferior peri-insular sulcus. Abbreviations in white letters denote sulci and fissures. ag, accessory gyrus; ahg, anterior Heschl’s gyrus; alg, anterior long insular gyrus; aps, anterior peri-insular sulcus; ar, ascending ramus of the sylvian fissure; ascs, anterior subcentral sulcus; asg, anterior short insular gyrus; cis, central insular sulcus; cs, central sulcus of Rolando; ds, diagonal sulcus; f2, inferior frontal sulcus; hr, horizontal ramus of the sylvian fissure; ia, insular apex; ips, inferior peri-insular sulcus; li, limen insula; msg, middle short insular gyrus; op, pars opercularis; or, pars orbitalis; pcg, precentral gyrus; pcis, precentral insular sulcus; pcs, precentral sulcus; pg, postcentral gyrus; phg, posterior Heschl’s gyrus; pis, postcentral insular sulcus; plg, posterior long insular gyrus; pmol, posteromedial orbital lobule; pog, posterior orbital gyrus; ps, postcentral sulcus; pscs, posterior subcentral sulcus; psg, posterior short insular gyrus; sis, short insular sulcus; smg, supramarginal gyrus; sp, sylvian point; sps, superior peri-insular sulcus; t1, superior temporal sulcus; T1, superior temporal gyrus; T2, middle temporal gyrus; tal, terminal ascending limb of the sylvian fissure; tdl, terminal descending limb of the sylvian fissure; tg, transverse insular gyrus; tp, temporal pole; tpl, temporal planum; tr, pars triangularis; tts, transverse temporal sulcus. Three opercular lips join together at the level of the sylvian fissure, closing the direct line of visualization to the insula. The fronto-orbital operculum is formed by the posterolateral aspect of the orbital gyri, but mainly by the pars orbitalis of the inferior frontal gyrus. It is delineated by the posterolateral part of the transverse orbital sulcus and the horizontal ramus of the sylvian fissure. This breach represents the edge between the fronto-orbital (anterior) and the frontoparietal (posterior) opercula. The temporal operculum follows a more clearly delineated forward course from the inferior part of the supramarginal gyrus toward the temporal pole, all along the superior temporal gyrus. The only constant indentation of this path is created by the connection of the transverse gyri of Heschl with the superior temporal gyrus (Figs. 11.8c, 11.9). Fig. 11.9 Artistic rendering of the left insular region (artificial retraction of the opercula) with detailed nomenclature. Abbreviations in white letters denote sulci and fissures. ag, accessory insular gyrus; ahg, anterior Heschl’s gyrus; aip, anterior insular point; alg, anterior long insular gyrus; aog, anterior orbital gyrus; aps, anterior peri-insular sulcus; ar, ascending ramus of the sylvian fissure; as, acoustic sulcus; ascs, anterior subcentral sulcus; asg, anterior short insular gyrus; atpg, anterior transverse parietal gyrus; atps, anterior transverse parietal sulcus; cis, central insular sulcus; cs, central sulcus of Rolando; ds, diagonal sulcus; fol, fronto-orbital limb; fos, fronto-orbital sulcus; gr, gyrus rectus; gs, gyri of Schwalbe; hr, horizontal ramus of the sylvian fissure; ia, insular apex; ips, inferior peri-insular sulcus; li, limen insula; log, lateral orbital gyrus; los, lateral orbital sulcus; mog, medial orbital gyrus; mos, medial orbital sulcus; msg, middle short insular gyrus; mtpg, middle transverse parietal gyrus; op, pars opercularis of F3; or, pars orbitalis of F3; os, olfactory sulcus; pcg, precentral gyrus; pcis, precentral insular sulcus; pcs, precentral sulcus; pg, post-central gyrus; phg, posterior Heschl’s gyrus; pip, posterior insular point; pis, postcentral insular sulcus; plg, posterior long insular gyrus; plol, posterolateral orbital lobule; pmol, posteromedial orbital lobule; pog, posterior orbital gyrus; pos, postinsular sulcus; ps, postcentral sulcus; pscs, posterior subcentral sulcus; psg, posterior short insular gyrus; ptpg, posterior transverse parietal gyrus; ptps, posterior transverse parietal sulcus; scg, subcentral gyrus; sis, short insular sulcus; smg, supramarginal gyrus; sopg, subopercular gyrus; sorg, suborbital gyrus; spcg, subprecentral gyrus; sps, superior peri-insular sulcus; ss, sulci of Schwalbe in the polar planum; stg, subtriangular gyrus; t1, superior temporal sulcus; T1, superior temporal gyrus; T2, middle temporal gyrus; tal, terminal ascending limb of the sylvian fissure; tdl, terminal descending limb of the sylvian fissure; tg, transverse insular gyrus; ti, temporal incisura; tos, transverse orbital sulcus; tp, temporal pole; tpl, temporal planum; tr, pars triangularis of F3; tts, transverse temporal sulcus. (From Türe U, Yaşargil DC, Al-Mefty O, Yaşargil MG. Topographic anatomy of the insular region. J Neurosurg 1999;90:723. Reprinted by permission.) Certain parts of these opercular segments are highly functional, representing some eloquent areas. The pars opercularis and the posterior segment of the pars triangularis of the frontoparietal operculum are frequently known as Broca’s speech area in the dominant hemisphere. The subcentral gyrus represents the junction of the precentral and postcentral gyri of the central lobe, and so it belongs to the primary motor and somatosensory areas. The inferior aspect of the supramarginal gyrus and sometimes the posterior part of the superior temporal gyrus are commonly termed Wernicke’s area in the dominant hemisphere, which is also related to speech functions. Finally, the transverse gyri of Heschl in the superior face of the superior temporal gyrus represent the primary auditory areas. Because of their high level of function, protecting these areas during insular tumor surgery is one of the challenges of these procedures. The insula itself has a pyramidal triangular surface delimited by the anterior, superior, and inferior peri-insular sulci. The limen insula is located at the anterobasal part of the insula, which consists of a narrow strip of olfactory cortex acting as the junction between the sylvian vallecula, the insula, and the fronto-orbital and temporal poles. The central sulcus of the insula separates the insula into anterior and posterior portions. The anterior insula is composed of three short insular gyri (anterior, middle, and posterior) and in some cases also by the transverse and accessory insular gyri. The transverse gyrus runs in a frontomedial direction toward the posteromedial orbital lobule, whereas the accessory gyrus joins the anterior portion of the anterior short gyrus with the suborbital gyri. The anterior and posterior short gyri are constant whereas the middle short gyrus is frequently found to be underdeveloped. These gyri join inferiorly to form the insular apex, which is the most superficial portion of the insula. The confluence of the anterior and superior peri-insular sulci coincides with the connection of the anterior short gyrus and the subtriangular gyrus at the so-called anterior insular point. The posterior insula generally contains two long gyri and is limited superiorly by the superior peri-insular sulcus and inferiorly by the inferior peri-insular sulcus, which join together at the posterior insular point (Figs. 11.8c, 11.9). A three-dimensional (3D) understanding of the architecture of the sulci and gyri of the medial aspects of the opercula is imperative. Their relationships to the insular surface remain constant and they may provide useful anatomic landmarks during surgical exploration. Two suborbital gyri (superior and inferior), located at the medial aspect of the fronto-orbital operculum, cover the anterior surface of the insula. The medial expression of the pars triangularis (sub-triangular gyrus), pars opercularis (subopercular gyrus), precentral (subprecentral and subcentral gyri), and post-central gyri (subcentral and anterior transverse parietal gyri), as well as the superior aspect of the supramarginal gyrus (anterior, middle, and posterior transverse parietal gyri) cover the superior surface of the insula. The polar planum (which consists of the sulci and gyri of Schwalbe), anterior and posterior transverse gyri of Heschl, and the temporal planum all together form the medial aspect of the temporal operculum and cover the anterior perforated substance and the inferior surface of the insula17 (Figs. 11.8b,c, 11.9). The subinsular region is composed of a sequence of layers of white matter and deep nuclei. Just underlying the insular cortex, the associated fiber system connecting the insula with the neighboring opercula is termed the extreme capsule. The claustrum is a thin layer of gray matter mainly located deep in the anterior insula just beneath the extreme capsule, and separates it from the external capsule. The external capsule is the theoretical edge of the subcortical insular tissue, and this capsule differentiates it from the next deep layer, which is composed of the lateral aspect of the putamen. Growing insular tumors push medially but respect the putamen, which is a wonderful deep anatomic landmark, especially in the central aspect of the insula.103 The insula receives its blood supply from the leptomeningeal branches of the M2 segment of the MCA.103 The M1 segment courses laterally within the sylvian vallecula all along the sylvian stem, and the lateral lenticulostriate arteries (LLAs) appear arising from this segment.14 This origin of the LLAs may represent a valuable landmark during tumor resection in the anteroinferior aspect of the insula (Fig. 11.10). The M1 segment turns approximately 90 degrees around the limen insula, forming the genu, where the main bifurcation occurs. This bifurcation has been highlighted as one of the main deep anatomic landmarks, and the surgeon must remember that, although in most cases it takes place at the level of the genu, it is also possible to find it distally or proximally.14 Early small branches arise from the bifurcation, or even proximal from M1, to feed the limen insula. Along the cortical course in the anterior insula, the inferior and superior M2 trunks give off several main branches before becoming M3. The lateral orbitofrontal artery supplies the anterior peri-insular sulcus and the accessory and transverse gyri. One of the most constant branches should be the prefrontal artery, which has been found in the anterior insular point, reaching the lateral hemispheric surface at the level of the sylvian point. The prefrontal, precentral, and central arteries usually extend small perforators to supply the anterior insula. The central artery runs along the central insular sulcus, which is the most vascularized region of the insula.14 The anterior and posterior parietal arteries usually supply the anterior long gyrus and some direct branches to the posterior short gyrus, whereas small perforators coming from the temporo-occipital, angular, and posterior temporal arteries feed the posterior long gyrus. At the anteroinferior aspect of the insula, the main blood supply comes from the anterior and middle temporal arteries (Fig. 11.10a). Approximately 96 (range 77–112) insular arteries arise from the M2 segment and supply the insula.14 These small and middle perforators must be identified during surgery, then coagulated and cut from their origin in the main branches to correctly devascularize the tumor. Careful attention must be paid to a special pattern of long perforators arising from the same M2 branches. These arteries have larger calibers, which are their specific features that extend as far as the corona radiata. These long perforators are mostly located in the posterior half of the central insular sulcus and on the long gyri, and special attention must be paid when tumor removal is performed at this level. Damage to these thin vessels may provoke severe corona radiata infarctions and consequent hemiparesis14 (Fig. 11.11). Fig. 11.10a,b Photographs of brain specimens. The insula is shown after removal of the frontal, parietal, occipital, and temporal lobes from the peri-insular sulci. The arteries of the insula originate from the M2 segment. (a) The insulo-opercular arteries (arrows) supply the insula and operculum. (b) Fiber dissection of this area reveals vascularization of the lentiform nuclei (which have been removed) and vascularization of the internal capsule by the lateral lenticulostriate arteries (LLAs; arrow), which arise from the M1 segment. 1, lateral orbitofrontal artery; 2, prefrontal artery; 3, precentral artery; 4, central artery; 5, anterior parietal artery; 6, posterior parietal artery; 7, angular artery; 8, temporo-occipital artery; 9, posterior temporal artery; 10, middle temporal artery; 11, anterior temporal artery; 12, temporal polar artery; ac, anterior commissure; alg, anterior long insular gyrus; asg, anterior short insular gyrus; cis, central insular sulcus; I, olfactory nerve; ia, insular apex; ic, internal capsule; it, inferior trunk of M2; M1, M1 segment of the MCA; msg, middle short insular gyrus; on, optic nerve; P2, ambient segment of the posterior cerebral artery; plg, posterior long insular gyrus; psg, posterior short insular gyrus; st, superior trunk of M2; tb, temporal branch of the MCA; te, tentorium. (From Türe U, Yaşargil MG, Al-Mefty O, Yaşargil DC. Arteries of the insula. J Neurosurg 2000;92:682. Reprinted by permission.)
Resection of Gliomas in Eloquent Areas of the Brain
Glioma Resection in an Awake Patient with Cortical Stimulation
Case Discussion
Clinical Presentation
Neurosurgical Management Paradigms
The Berger-Sanai Insular Glioma Classification System
Outcomes Analysis
Conclusion
Optimal Resection of Insular Glioma with Image-Guided Technologies
Technological Advances in Glioma Surgery
Patient Evaluation
Treatment Strategy
Surgical Approach
Surgical Anatomy of the Insula
Complementary Imaging
Detecting Anaplastic Foci
Operative Management
Discussion
Glioma Resection in Eloquent Areas: Anatomical Basis and Resection with Tractography Studies
Low-Grade Gliomas in Eloquent Areas
The Insula: Anatomic Considerations
The Sylvian Fissure
The Opercula
The Insular Surface
The Insula as It Relates to the Opercula
Subinsular Anatomy
Vascularization
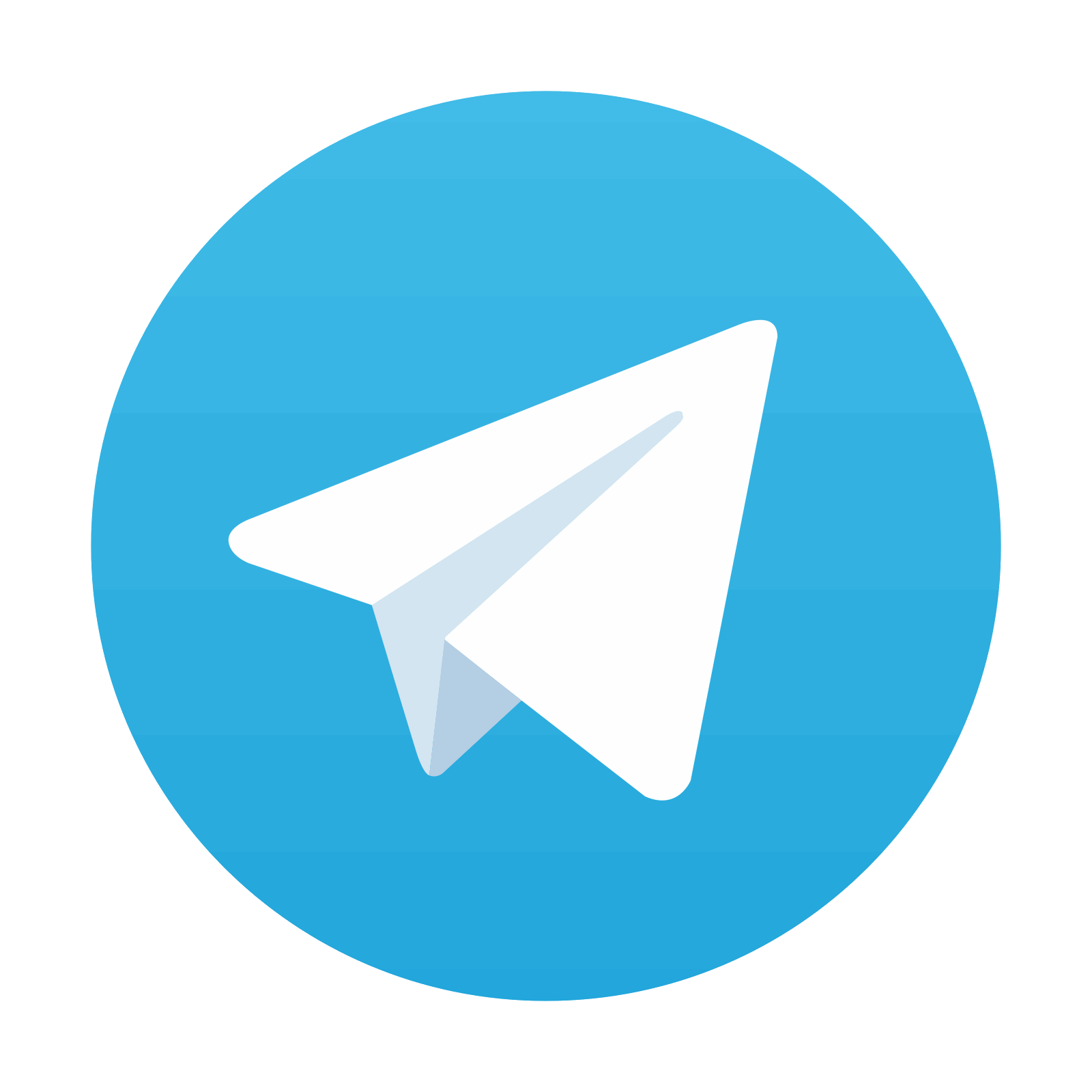