INTRODUCTION
Epidemiology provides the basic tools and framework for understanding the distribution and risk factors for disease in human populations. Identifying and describing the population distribution and characteristics of people who have Parkinson’s disease (PD) bring us closer to solving the mystery of what causes the disease. Epidemiologic research must be conducted rigorously in order to reach valid conclusions. PD, in particular, presents many challenges to epidemiologic study.
Because there is no diagnostic test for PD, misdiagnosis may be common in some settings. Disorders such as essential tremor, atypical parkinsonism, or secondary parkinsonism may be misclassified as PD, and cases identified through nonneurologist sources can overestimate the disease by 40% or more (1–4). Differential access to neurologist specialist medical care can impact apparent rates of PD. The source of diagnostic information and its potential for underascertainment or misdiagnosis must always be considered. Even experienced examiners can misdiagnose PD early in the course of disease, when distinguishing features of other disorders may not yet be present. Comparisons across studies are hampered not only by differences in the experience of the diagnostician and individuals’ access to care but by differences in the study diagnostic criteria. These may vary greatly over time, but can also be quite different among contemporaneous studies (5–7).
In addition to challenges related to accurate and complete case ascertainment, PD presents particular challenges for etiologic–epidemiologic studies. Because it is primarily a disease of old age, many individuals with early or mild PD die from other causes before motor symptoms manifest, obscuring risk factor associations (8,9). Furthermore, pathologic evidence of a slowly progressive neurodegenerative process suggests that disease-associated environmental exposures may occur years or even decades before diagnosis, making them extremely difficult to identify retrospectively. Finally, considerable variability in clinical course, disease features, and pathology suggest etiologic heterogeneity.
The relative strengths and weaknesses of the study design, and appropriateness to the research question, must also be considered when interpreting the results of epidemiologic studies. For example, observations are most informative when they apply to all cases of disease within a population, referred to as complete ascertainment. Because persons missed may be different from those identified, incomplete ascertainment may lead to false or misleading conclusions, and represents a possible source of bias in epidemiologic studies. Cases identified using health utilization records or registries (e.g., pharmacy records, billing databases, Medicare claims, death records) may better reflect the overall population, but these studies may suffer from poor diagnostic accuracy and low sensitivity, especially among certain demographic strata, and miss those with no access to medical care (10–12). PD cases identified through specialty clinics have high diagnostic validity, but may differ from the majority of individuals with PD who do not attend tertiary referral clinics. For example, in the United States, specialty clinic patients tend to be younger, male and Caucasian, are more likely to have a family history of PD, and are better educated and from higher socioeconomic strata. Therefore, study findings based on specialty clinic populations may not be generalizable to the broader population (13,14). The third approach is to screen a defined population. Examples of this include door-to-door studies, which attempt to identify all affected persons within a defined geographic area, and prospective cohorts comprised of unaffected individuals who are followed over time until some proportion develop disease. Door-to-door studies are the gold standard for incidence and prevalence studies, because they have high diagnostic sensitivity and validity, and are able to ascertain previously undiagnosed cases. Prospective cohort studies are the gold standard for assessing risk factors, because information on putative risk factors is collected prior to disease onset, thereby minimizing recall bias and possible reverse causation. Because PD is relatively rare, door-to-door and prospective cohort studies must screen and/or follow huge numbers of persons at great expense and over long time periods. For this reason, virtually all prospective cohorts studied for PD were initially established to study other more common disorders such as heart disease and cancer, and therefore, information on putative risk factors for PD is usually quite limited.
DESCRIPTIVE EPIDEMIOLOGY
Descriptive epidemiology considers disease frequency in populations and population subsets. By understanding how PD frequency varies with respect to demographic factors such as age, gender, race, geographic location, and time, descriptive epidemiology can provide important clues regarding disease causation, as well as generate information for health-care resource utilization and planning. When interpreting descriptive epidemiologic statistics and comparing results among studies, it is essential to consider the specific characteristics of the population studied as well as the methods used. PD is a disease of aging, and rates may vary between genders and among racial or ethnic groups. Standardization to a reference population allows comparison of the rates of disease among populations with different demographic characteristics.
INCIDENCE
Incidence is the number of new cases of a disease occurring in a specific population during a given period of time. It is the best measure of disease frequency, because it is not affected by survival after diagnosis or by migration of affected individuals. For PD, estimated crude incidence ranges from 5 to 20/100,000/year in different reports (6,15,16). Because the demographic characteristics of these populations differed, as did case ascertainment methods and diagnostic criteria, it is possible that this fourfold difference may in part reflect these factors, rather than a true difference in disease incidence. In studies using uniform criteria and similar methods, incidence rates are more closely aligned (Table 5.1).
PREVALENCE
Prevalence is the total number of individuals in a population who have disease at a specific point in time. Prevalence reflects both incidence and survival. Prevalence increases with improved treatments and longer survival, even if incidence remains constant, as suggested for PD patients in a Canadian study spanning 20 years (17). For this reason, prevalence is most useful as a metric for health-care planning and resource allocation, and less valuable for etiologic inference. Studies that report prevalence in age-specific strata are shown in Table 5.2. As for incidence, adjusting values to a standard reference population yields a much narrower prevalence range (17).
The prevalence of PD is increasing dramatically as life expectancy has increased. The number of cases worldwide is expected to double to approximately 9 million by 2030 (18). Disease-related annual costs in the United States are estimated at $14 to $23 billion, and a projected $50 billion by 2040 (19,20).
MORTALITY
Because PD is not a direct cause of death, the diagnosis is often not reported on death certificates, even as an underlying or contributing cause, with 40% to 60% of cases missed. Studies based on death certificates may underestimate mortality, and underreporting may be greater in certain populations (21–23). Prospective cohort studies that follow patients until death provide more reliable data on PD mortality. Reported mortality rates relative to the general population vary over time and place. Threefold increased mortality has been suggested in some older studies (24–26). In more recent years, rates comparable to the overall population have been reported in some populations, perhaps due to improved treatment and access to care (23,27–29), but this finding is not universal, and increased mortality continues to be reported in some populations or subgroups (30–33). Because population demographic characteristics, and cultural and socioeconomic factors may differ among these populations, differences in mortality might be expected. Clinical and demographic characteristics associated with shortened survival include male sex, lack of tremor, predominance of postural instability and gait disorder, symmetry of motor signs, cognitive impairment or dementia, hallucinations, and poor response to dopaminergic therapy (23,29,34–38).
SPECIFIC POPULATION CHARACTERISTICS
Age
PD increases with age. Incidence in persons aged 80 or older may be 1,000 times higher than in persons under age 40 and 10 times higher than incidence in those in their sixth decade (see Table 5.1) (12,25,26,39,40). Decreased incidence in the oldest age groups has been reported (26,41–44). Rather than representing a true drop in incidence, this could reflect incomplete ascertainment of PD in the very old, due to limited access to specialty care, confounding comorbid conditions, and imprecise estimates, due to the small number of persons in the oldest age groups. In many populations, PD incidence continues to increase with increasing age (39,43,45–50). The association between increasing age and PD incidence may reflect an age-related neuronal vulnerability or a causal mechanism dependent on the passage of time (51).
Gender
Incidence and prevalence of PD is higher in men in most populations worldwide (15,39–43,45,47–49). For example, incidence was approximately twofold higher in men than in women in studies in Northern California, Italy, Scotland, and Argentina that used thorough ascertainment methods (40,45,47,48). The reason for a greater risk of PD in men is unknown, but may be due to protective effects of female sex hormones (see Section III.C.2.) or greater male exposure to risk factors such as occupational toxicants or head injury (see Section III.B.3.a.). Differences in the male:female ratio across populations and across time provide some support for this possibility (42). Alternatively, a male preponderance could also suggest a sex chromosome–linked genetic predisposition.
Reported prevalence has shown more variability among populations. Most studies report greater prevalence in men (15,39,41,42), but a few studies found similar prevalence in men and women (10,12,16,52–54), and in Japan, PD prevalence is consistently higher in women (55–57). These differences could be an artifact, due to longer survival among females in Japan, differential access to medical services, or other underascertainment of male cases. However, because a female preponderance was observed in several cities over decades, an actual, unexplained excess prevalence in women is suggested.
Race and Geography
PD incidence may vary by race or ethnicity, but this has been addressed systematically in only a few studies. Prevalence studies—subject to more potential bias—also show variation by race and ethnicity. Disparities may suggest actual differences in PD risk due to inborn (genetic) and/or acquired (environmental) susceptibility factors, although differences in study methods or access to care must also be considered.
Persons of African or Asian descent may have lower risk than persons of Caucasian or European origin. Only a few studies have determined race-specific incidence using uniform methods. Incidence of PD in a large, multiethnic health maintenance organization in Northern California was highest among Hispanics, followed by non-Hispanic Whites, Asians, and Blacks (40). Although race-specific estimates were relatively imprecise, all members had equal access to health care, and broadly reflected the underlying racial and ethnic composition of the region, reducing the likelihood of ascertainment bias. A serial cross-sectional study of US Medicare beneficiaries aged 65 and older from the years 1995 and 2000 to 2005 also found PD to be more common in Whites (58).
Incidence and prevalence estimates also vary widely by country (see Tables 5.1 and 5.2). Twelves et al. found adjusted incidence in some countries to be more than twice that reported for others (from 8/100,000 in Italy to 18/100,000 in the United Kingdom) (6). Prevalence varies even more widely, ranging from 18/100,000 in China to 234/100,000 in Uruguay (59). Reported rates are low in Sardinia, Italy, and Benghazi, Libya (15,53), but are high at extreme northern latitudes. Age-adjusted prevalence in Inuit populations of Greenland and the Faroe Islands was double that of Denmark and Norway (60–63). Geographic differences are not easily separated from race, and, as discussed above may reflect socioeconomic or cultural differences rather than true differences in rates.
Studying disease rates among members of a racial/ethnic group in different geographic locations may help to elucidate risk factors. For example, in men of Japanese and Okinawan origin living in Hawaii, incidence of PD was similar to that of Caucasian men in Europe and the United States, and higher than incidence rates published for Asian men living in Asian nations (25,26). Although differences in study methods are a possible contributing factor, this geographic variation in persons of the same race may be due to environmental differences between Hawaii and Japan. Similar geographic variation has been observed among persons of African descent, although only a handful of studies have been conducted in sub-Saharan Africa (64). A study in Copiah County, Mississippi, used door-to-door ascertainment to minimize bias due to racial differences in access to care. Prevalence of PD was similar in African Americans and Whites when the least stringent diagnostic criteria were used to define PD (10). In Nigeria, using the same door-to-door method, age-adjusted prevalence was 67/100,000, compared to 341/100,000 for African Americans living in Mississippi (65). More recently, Dotchin and colleagues used door-to-door case-finding methods in rural Tanzania, and found age-adjusted PD prevalence to be only 40/100,000 overall (66). Of note, only 8 of 33 patients identified in this study had been previously diagnosed, emphasizing the importance of the door-to-door methodology in underserved areas.
These findings suggest that PD risk may vary by both race and geography. Differences may be due to genetic causes, as in LRRK2-associated PD seen in Israel (67), or to environmental causes, as is suggested by the differences in disease frequency between those of the same race living in different environments.
Calendar Time
If the incidence of PD changes over time, this could be due to a change in exposure to environmental risk factors for the disease, such as those associated with industrialization. Changes in disease frequency are difficult to study because of changes in diagnostic criteria and acumen. They can be explored in only a few locations where accurate incidence and prevalence estimates are available for extended periods of time. The population of Olmsted County, MN, has been studied for the longest period (16). Incidence gradually increased from 9.2/100,000 for the interval 1935 to 1944 to 16.3/100,000 for the interval 1975 to 1984 (68); however, the actual number of incident cases was few, and precision was poor. Recently, Savica et al. identified a total of 464 incident PD cases from 1976 to 2005 (69). After age adjustment to a standard population, incidence in men increased from 18.2 in 1976 to 1985 to 30.4 in 1996 to 2005 (trend p value = 0.0005). In contrast, the age-adjusted incidence of PD in women did not change. Mirroring this gender-specific observation, a Finnish study found no overall change in incidence between 1971 and 1992, but saw a 50% increased rate in men and a 30% decreased rate in women (70). One possible explanation is that incidence estimates were falsely inflated earlier in the period due to nonspecific diagnostic criteria, as reflected in the decreasing rate in women, while in men there was a true substantial increase in incidence, due to increasing environmental risk factors.
Estimates of PD prevalence in mainland China based on door-to-door ascertainment methods found rates much lower than those reported in western countries (71,72). More recently, studies in Taiwan and Beijing found prevalence to be much closer to that of Western countries (see Table 5.1) (25,73,74). Because environmental factors have been changing rapidly in China over the last several decades, further studies of these populations could provide important insights. Similarly, in two Japanese communities, service-based and door-to-door studies also found increased prevalence over a two-decade interval (57). Although the study authors believe that improved ascertainment explains this difference, an actual change in prevalence is equally possible.
ANALYTIC EPIDEMIOLOGY
Examining the association of specific characteristics and PD can provide clues to the causes of disease. Many factors have been associated with an increased or decreased risk for PD (Tables 5.3 and 5.4). The causes of PD are likely to be multifactorial, a combination of age-related, genetic, and environmental risk factors.
FAMILIAL AND GENETIC ASSOCIATIONS
Familial Associations
If PD occurs more commonly in persons with affected family members, this could implicate genetic factors. Ninety percent of PD patients have no family history of PD (75), although families with multiple affected individuals are more likely to come to the attention of specialty clinics. Community-based studies find a 1.5- to threefold increased risk of PD in persons with an affected first-degree relative (75–77). Familial aggregation could reflect shared environments as well as shared genomes. Twin studies also provide clues to the relative contribution of genetic factors to the cause of disease. If the cause of a disease is primarily genetic, then the rate of disease in monozygotic (MZ) twins of affected twins should be greater than that in dizygotic (DZ) twins, but two large, population-based twin studies showed similar rates of concordance for PD in MZ and DZ twin pairs (78,79), arguing against a primary genetic cause for most PD.
| Factors Associated with Increased Risk for Parkinson’s Disease |
Demographic factors
Increasing age
Male sex
White race
Family history of Parkinson’s disease
Lifestyle
Head trauma
Diet (dairy products, animal fats)
Biometric and comorbid conditions
Lower blood cholesterol
Lower serum uric acid
Oophorectomy
Environmental exposures
MPTP
Pesticides (paraquat, rotenone, 2,4-D, dieldrin, maneb)
Industrial agents (PCBs)
Solvents (TCE, PERC)
Metals (lead, iron)
Rural residence
Drinking well water
Farming
Pulp mills
Occupation (health care, teaching, woodworking/carpentry, legal work, religious work)
Infection
| Factors Associated with a Decreased Risk of Parkinson’s Disease* |
Cigarette smoking and tobacco use
Coffee and tea drinking
History of gout
Diet
“Mediterrenean” diet
Polyunsaturated fats
Uricogenic diet
High physical activity
Medications
Calcium channel blockers
Nonsteroidal anti-inflammatory drugs
Statins
*Not including the inverse of factors in Table 5.3.
Genetic Associations
The first monogenic cause of PD, a point mutation in the gene encoding α-synuclein (SNCA), was identified in 1997 by Polymeropoulos and colleagues (80). Highly penetrant Mendelian forms of PD have now been associated with mutations in at least seven genes, but in most populations, causative mutations in these genes have been found in fewer than 5% of patients (80,81). In contrast to the rare highly penetrant genes, genome-wide association studies (GWAS) have identified at least 28 variants across 24 loci that more modestly influence PD risk (82).
Increasingly, the influence of both genetic predisposition and environmental exposures is recognized as the likely cause of disease in most cases. Identification of gene–environment interaction is dependent on having information on genetic and environmental factors as well as PD diagnosis in the same individuals. This information is available in relatively few populations. Examples of gene–environment interaction are provided in the sections below. Chapter 20 provides a detailed review of PD genetics.
ENVIRONMENTAL ASSOCIATIONS
Introduction
Interest in environmental causes of PD was ignited in the 1980s by the identification of a cluster of cases of parkinsonism produced by 1-methyl-4-phenyl-1,2,3,6-tetrahydropyridine (MPTP), a simple pyridine compound that induces most, if not all, of the features of PD in humans (83) and experimental animals (84). Although MPTP is rare outside of the laboratory, related chemicals more commonly present in the environment were proposed as possible causes of PD. Epidemiologic studies have identified and investigated numerous associations between environmental exposures and PD. The sections below are organized by the direction (direct or inverse) of the association of the environmental factor and risk of PD. One must bear in mind that “association” is not synonymous with etiologic “causation,” which can only be established through replication of a biologically plausible association in multiple high-quality studies (85).
Environmental Factors Associated with Reduced Risk of PD
Smoking. An inverse association of cigarette smoking with PD risk has been consistently observed in numerous studies worldwide over several decades. A meta-analysis that pooled results from four-dozen case–control and cohort studies found a 40% reduced risk for ever smoking (86). A dose–response relationship was observed, with a 15% to 20% reduced risk per each 10 pack-years smoked. These results have been repeatedly confirmed, including a discordant twin study (78) and a carefully conducted pooled analysis of individual-level data from 11 studies (87). Interestingly, the risk reduction from passive exposure to cigarette smoke was found to be comparable to that of active smoking (88). In addition, heavy lifetime smoking was also associated with reduced nigral Lewy pathology, in a community-based prospective cohort (89).
Gene–environment interaction. The inverse relationship with smoking may be modified by genetic polymorphic variants, including NOS2A (nitric oxide synthase, inducible), MAO-B (monoamine oxidase B), SNCA (α-synuclein), GSTs (glutathione-S-transferases), NAT2 (n-acetyltransferase 2), and LRRK2, although data are limited and replication rare (90–96).
Caffeine/Coffee drinking. Coffee drinking or caffeine intake is another behavior inversely associated with the risk of PD (86,97–101). As with smoking, this association has been observed in dozens of studies worldwide, with a clear dose–response relationship and an approximate 40% reduction in PD risk among regular coffee and tea drinkers. In a compelling study with 30 years of follow-up, the incidence of PD decreased from 104/100,000 person-years in men who drank no coffee to 19/100,000 in those who drank at least 28 ounces per day. Notably, this association persisted after adjustment for cigarette smoking (99). Similar results were reported in other large prospective cohorts (98,101–103). Results are similar when analyzed by total caffeine consumption, and in populations that are predominantly tea drinkers (104–106). No such relationship has been observed for consumption of decaffeinated coffee (98,102).
Several studies reported a stronger inverse association among men, although meta-analyses find no consistent gender-specific effects overall (101). However, Ascherio et al. reported an interesting difference among women, that caffeine reduces the risk of PD only among women who do not use postmenopausal hormones (107). This finding suggests a potential interaction between caffeine and estrogen and is consistent with data from animal studies (108). Neuroprotective effects of caffeine may be mediated in part through its antagonist action on the adenosine A2 receptor (109,110).
Gene–environment interaction. The inverse association of caffeine and PD may be modified by genetic variants, including the xenobiotic metabolizing enzyme CYP1A2 (cytochrome P-450 1A2) (111–113), ApoE (apolipoprotein E) (93), and GRIN2A (glutamate receptor, ionotropic, N-methyl-D-aspartate [NMDA] 2A), which encodes an NMDA–glutamate-receptor subunit that regulates excitatory neurotransmission (114,115).
Medications
Nonsteroidal anti-inflammatory drugs. Inflammation is a primary mechanism implicated in the nigral degeneration found in PD (116), and this has prompted numerous epidemiologic investigations of the use of nonsteroidal anti-inflammatory drugs (NSAIDs) and PD. A lower incidence of PD in those who use NSAIDs has been reported in many studies (117–123), although two studies showed little or no association (122,124). NSAID associations differ for specific compounds. During 6 years of prospective follow-up among 136,197 participants in the Nurses’ Health Study (NHS) and the Health Professionals Follow-up Study (HPFS), use of ibuprofen at baseline was significantly associated with a reduced risk of subsequent PD (OR 0.62, 95%; CI 0.42–0.93), with a significant dose–response relationship for tablets taken per week (125). In contrast, risk was not related to the use of aspirin, other NSAIDs, or acetaminophen. Similar results were found in the Cancer Prevention Study II Nutrition cohort (126) as well in meta-analyses (125,127), suggesting a protective effect specific to ibuprofen. A possible gender-specific effect was observed in several studies that found a stronger inverse association with NSAIDs in women (123,126).
Calcium channel blockers. Calcium channel blockers reduce apoptosis and glutamate-mediated excitotoxicity, and confer resistance to MPTP in animal models (128–130). These observations prompted epidemiologic investigations of calcium channel blocking medications in human populations. Studies in humans have been inconsistent. Two registry-based studies found a 30% lower risk of PD in persons taking dihydropyridine calcium channel blockers (131,132), although one study found this effect only in current rather than in former users (132), and no dose–response relationship was seen in either study. Similarly, a study in the General Practice Research Database found an association only among current users and no dose–response, suggesting possible confounding (133). However, several studies that found an inverse association with calcium channel blocker medications found little association with other antihypertensives (131,133,134). In contrast, no association was seen in a population-based case–control study (135) or in the large prospective NHS and HPFS studies (136).
Although an inverse association with calcium channel blocker medications could reflect reverse causality, that is individuals with early PD might be less likely to need antihypertensive treatment, the biologic plausibility and the fact that other antihypertensive medications have not been associated with a reduced risk argues for a specific protective effect of calcium channel blocking agents.
Statins. The use of cholesterol-lowering statins (HMGCoA reductase inhibitors) has been associated with a reduced risk of PD in a number of studies. The significance of this finding is difficult to parse out from the relationship of PD and serum cholesterol, which has been linked with decreased PD risk in prospective cohorts (137,138). In addition, results for specific statins have varied, possibly reflecting differences in brain penetration or potency (139).
Several case–control studies found a reduced risk of PD associated with statin use (140,141). In a survey of US Veterans Affairs administrative databases, PD risk was lower in users of simvastatin (hazard ratio [HR] 0.51, 95%; CI 0.4–0.55), but not atorvastatin or lovastatin (142). A large prospective study in Taiwan found that continuation of either of the lipophilic statins simvastatin or atorvastatin was associated with markedly lower risk in comparison with individuals who discontinued statin use (143). Gao and colleagues found a 25% reduced risk in the NHS and HPFS cohorts (144), and similar results were reported in a prospective Israeli study (145). Interestingly, this latter study found similar inverse associations with statin use across strata of baseline LDL-C cholesterol, suggesting that the relationship with statin use was not confounded by cholesterol levels. However, other prospective studies did not see an association with statins (146,147).
Environmental Factors Associated with Increased Risk of PD
Head injury. The association between head trauma and PD has been investigated in many case–control studies and several registry-based prospective studies (Fig. 5.1). Many studies reported a positive association between head trauma and PD risk (148–159), and higher risk with more frequent injuries (152–154,159); others, however, found no relation between head trauma and PD risk (160–166). Because most studies relied on the report of the person with PD or a control, biased recall has been a concern, although studies using head trauma documented in databases have also shown differing results (152,163). Another important concern is the possibility that early, undiagnosed disease increases the risk of falling and sustaining a head injury. Consistent with this possibility, some studies found attenuation of the association when they excluded injuries that occurred in close temporal proximity to diagnosis (163,167). However, head injury may initiate a pathologic inflammatory cascade that takes years to evolve, and studies that do not ascertain head injuries that occur in childhood and adolescence might underestimate an association.
Gene–environment and environment–environment interactions. In addition to methodologic differences, inconsistencies across populations might be explained by genetic susceptibility to the effects of head injury, or to differences in exposure to other environmental risk factors. Goldman and colleagues found that head injury was not associated with PD risk overall, but in individuals with a SNCA variant associated with higher levels of α-synuclein protein, head injury was associated with a 3.5- to sixfold increased risk and an earlier age at onset (168). Risk associated with head injury was also found to be markedly greater in individuals exposed to the herbicide paraquat (169).
Occupation. A higher frequency of PD has been reported in numerous studies of occupational titles or job categories. An increased risk in teachers has been reported with high consistency in mortality databases (170–173), although case–control studies are less consistent (174–178). Disease frequency in health-care workers, especially physicians, is similarly elevated in many studies (171,175–177,179). Infection has been proposed as one possible explanation for these observations, as has a “premorbid” altruistic personality that might predispose individuals to choose these careers (180). Alternatively, the frequency may only appear to be higher due to more complete ascertainment. Other white-collar job categories that have been associated with an increased risk of PD in multiple studies include legal, religious, scientific, and administrative occupations (171–173,175,176,181). Few blue-collar occupations have been associated. This may partly result from a “healthy worker effect,” whereby healthy individuals are overrepresented in physically demanding jobs, or because of greater physical activity, which has been associated with reduced risk of PD. Elevated risk is consistently seen for woodworking jobs (149,171,173,178,179,182). Risk was also markedly increased in female laundry and dry cleaners in a Danish health-system-census-linkage study (181), and a Swedish case–control study (182), although numbers were small. A hypothesis for increased risk among woodworkers and cleaners is exposure to solvents (see Solvents).
A relationship between welding and PD has been highly controversial (183). In a clinic series and an occupational group referred for medicolegal evaluation, welding was associated with higher prevalence of PD (184,185); however, welding has not been associated with PD risk in other studies using clinic- or industry-based samples or in studies linking occupational and disease registries (186).
Agricultural and farming-related jobs comprise the occupational category most robustly associated with PD risk. Associations with pesticides are discussed below.
Pesticides. PD may be more common in rural residents, and farmers may be at increased risk (187–189). These observations, and the recognition that several commonly used pesticides resemble MPTP structurally or mechanistically, spurred numerous studies of pesticide exposure and PD risk. Pesticide exposure was associated with a doubling of risk in a meta-analysis of 19 studies worldwide (190), and a similar increase was found in a more recent meta-analysis that included 51 case–control studies (OR 1.76, 95%; CI 1.56–2.04) (191). Most studies assessed pesticide exposure in a very general way, such as by functional class (i.e., insecticide, fungicide, herbicide), limiting etiologic inference. Far fewer studies have assessed risk associated with specific pesticides.
Rotenone. Rotenone is a plant-derived compound that has been used as a pesticide for centuries. In modern times, it has been used as an insecticide in agriculture and home gardening, in household pet products, and as a piscicide to eradicate invasive fish species in lakes (192). Like MPTP, rotenone inhibits mitochondrial Complex I (193,194), and in animal models, it selectively damages nigral dopaminergic neurons (195). Tanner and colleagues found a significantly increased risk of PD associated with rotenone use in a case–control study nested in an 80,000-person cohort of licensed pesticide applicators and their spouses (OR 2.5, 95%; CI 1.3–4.7) (196).
Paraquat. Paraquat is a bipyridyl herbicide used since 1962 as a defoliant and preemergence dessicant (197). It is a structural analog of MPTP and produces an animal model of parkinsonism (198). At least five case–control studies found increased PD risk associated with exposure (178,196,199–201), while two found no association (177,202). In particular, risk was increased 2.5-fold in a large study of pesticide applicators (196), and in a study that used geographic mapping linked to pesticide application data.
Gene–environment interaction.
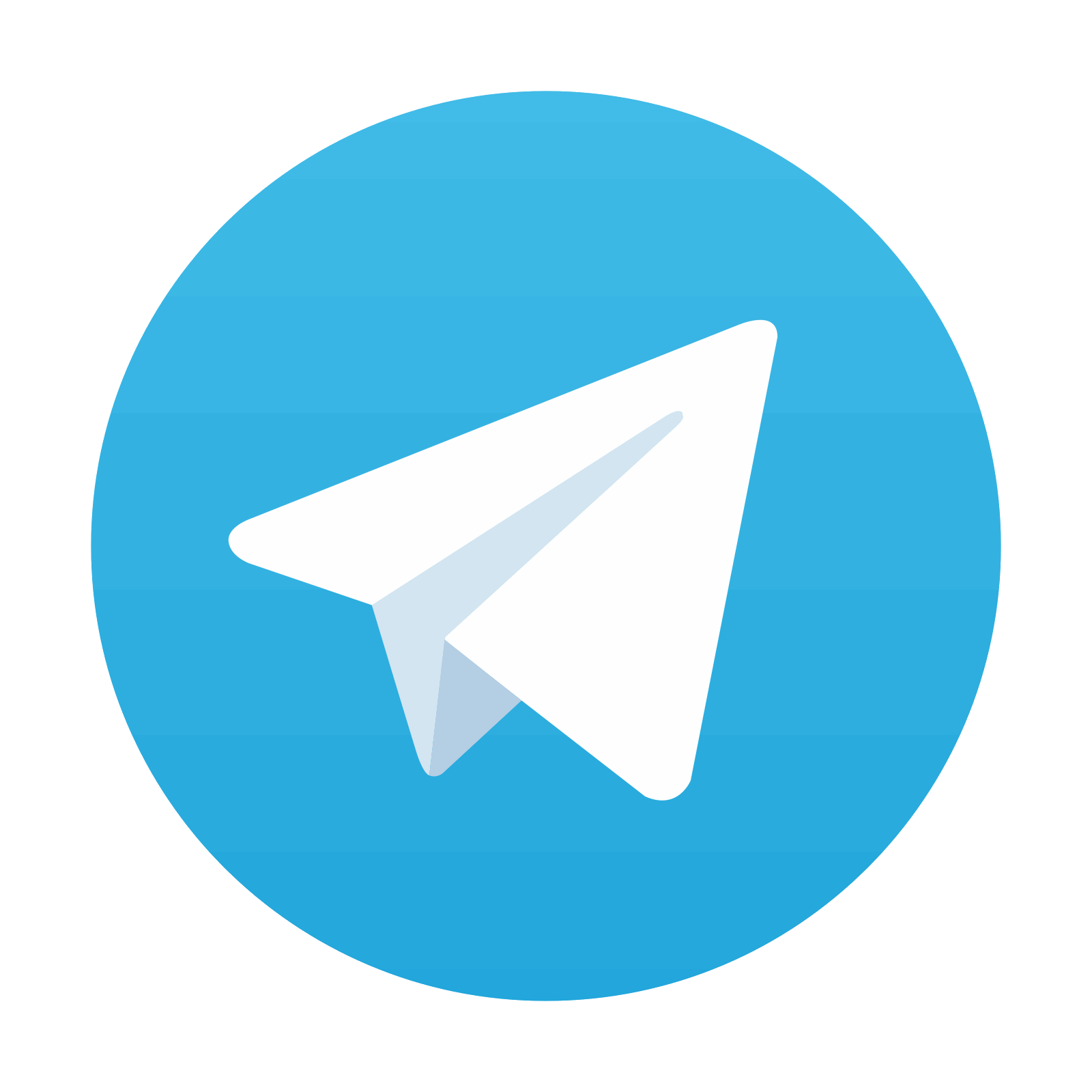
Stay updated, free articles. Join our Telegram channel

Full access? Get Clinical Tree
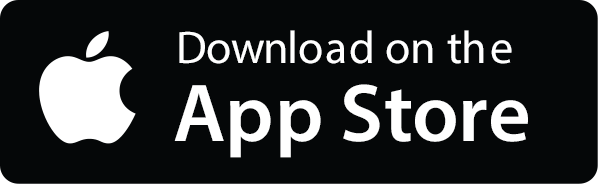
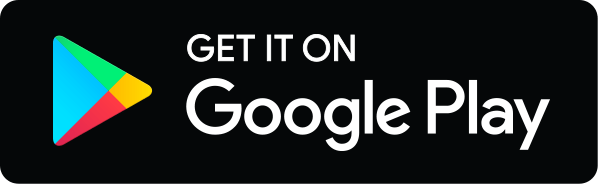