Fig. 44.1
Progression of scoliosis with growth from a straight spine (a), with disk wedging (b), and finally vertebral wedging (c). If the deformity is treated with a tether placed on the convex side to limit growth (d), disks straighten (e), then finally vertebrae grow straight (f). Once the tether is removed or cut, the spine has theoretically returned to a normal straight spine. A similar effect may be seen in the sagittal plane
44.1.2 Spinal Growth Modulation Theory Overview
Spinal growth modulation provides an important alternative to treat spinal deformity in the young child [14]. As naturally occurring animal models with spinal deformity are not available, growth-modulating techniques are used to either correct iatrogenic deformities created in animal models [1, 15–18] or are used to create a deformity in a straight spine [19–26]. Scoliotic animal models created by posterior spinal tethering or rib tethering may not best mimic the human condition as the disks and growth cartilage have already experienced unusual forces prior to application of the growth-modulating treatment. Spinal growth modulation to create a scoliotic deformity from a normal immature animal spine may in fact be more similar to the clinical condition and more appropriate to the study the responses of the vertebral body and intervertebral disk to the forces created by growth modulation (Fig. 44.2a–d).
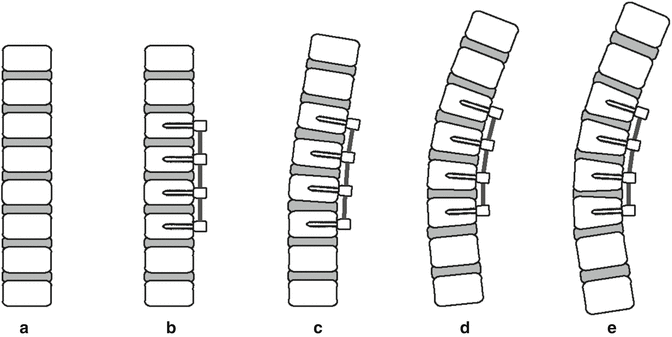
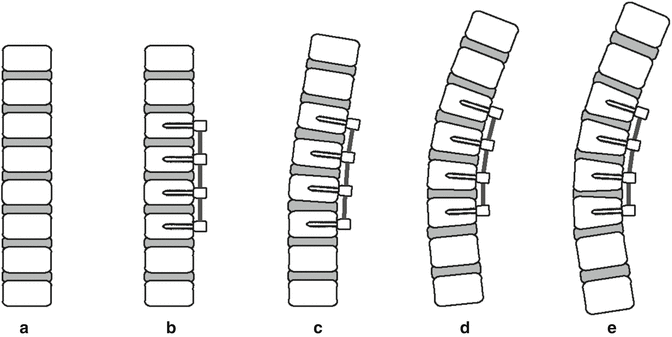
Fig. 44.2
Experimental growth modulation starting with a straight animal spine (a). A tether to growth is placed unilaterally (b), first resulting in deformity of IVD (c), then VB wedging with straightening of the IVD (d), and finally, VB wedging to create deformity with a metabolic response of the disk which results in “reverse” wedging of the disk (e)
44.2 Growth Modulation Applications in the Spine
Nonsurgical applications of growth modulation of the spine (casting and bracing) have regained popularity recently. Bracing may be used for smaller curves as a means of loading the spine and allowing controlled distribution of forces within the spine, thus modulating growth if worn during a growth spurt. [27] The efficacy of bracing has been established by a multicenter randomized cohort by Weinstein et al., and the trial was stopped early due to the documented efficacy of bracing [28]. However, the effectiveness of brace wear is strongly dose related, and there are clear limitations of brace use related to compliance of wear and in the selection of which patients actually benefit from brace wear.
Surgical methods that do not involve the use of implants, such as convex side costoplasty to treat the cosmetic deformity of the posterior rib hump, have provided evidence for the effect of rib asymmetry in the pathogenesis of idiopathic scoliosis [29]. Experimental studies have shown that scoliosis can be corrected by rib length modulation (shortening or lengthening), taking into account the growth potential of the spine and rib cage [30–32]. These procedures, however, have yet to gain wide acceptance, as their biomechanical effects on the spine are not well understood, and other treatment options are increasingly available. A unilateral fusion, often termed a hemiepiphyseodesis, as a means of altering spinal growth has been used primarily in congenital scoliosis but may have a limited use in other forms of childhood scoliosis [33]. The technique has had mixed outcomes, but in some cases, deformity correction has been noted (if the age is less than 5 years, deformity less than 50°). The downside of this approach is the loss of motion associated with the arthrodesis, the loss of future growth, and the relatively low reliability of true growth modulation with the technique. Such a hemiarthrodesis likely acts as a boney tether to alter spinal growth.
Asymmetrical growth at the neurocentral synchondrosis (NCS) has also been considered as another possible etiology for the three-dimensional spinal deformity seen in AIS. In theory, an abnormality at the neurocentral junction is thought to produce pedicle length asymmetry, thus leading to vertebral rotation [34]. Vertebral wedging is then thought to result from rotation-induced increases in compressive loads on the vertebral growth plates [35]. Once the initial deformity is present, scoliosis is thought to develop due to the propagation of asymmetric loads by the Hueter–Volkmann effect. Theoretically, altering this pattern could halt progression and may provide a means for correction. Previous anatomic studies of normal and scoliotic vertebrae have found an association between a longer pedicle and vertebral rotation [36–38]; however, a causative relationship could not be established. A 2007 biomechanical simulation with asymmetrical pedicle geometry was not able to produce significant scoliosis, vertebral rotation, or wedging [39] and concluded that asymmetry of pedicle growth rate alone was not sufficient to cause scoliosis. On the other hand, asymmetric epiphysiodesis of the NCS was performed in a growing porcine model and was able to create a scoliotic deformity with axial vertebral rotation with the magnitude of the deformity created correlating with the degree of NCS physis closure [40].
44.2.1 Growth Modulation: Anterior Implants
Anterolateral implants are now being used as an internal brace (no patient wear compliance issues) to limit curve progression and ideally improve the deformity during the patient’s remaining spinal growth. Such treatment may delay or eliminate the need for a definitive fusion procedure. In theory, the implants could be removed at maturity, leaving the patient with a “normal” spine with full flexibility and function.
Early attempts to provide such an internal brace used vertebral body staples, applying principles learned from growth modulation of the long bones as described in 1951 by Nachlas and Borden [41]. However, unlike the situation with long bones, the vertebral staples were required to cross a joint (intervertebral disk), and loosening of the implants was a common concern. Advancements in staple design, primarily the use of a temperature-dependent shape memory metal (nitinol), have led to more promising clinical results in terms of safety and efficacy to stabilize or modulate growth in moderate-sized curves [1, 15, 42–47]. A biomechanical study evaluating spinal flexibility after instrumentation with nitinol staples found that they staple significantly restricted motion, especially in axial rotation and lateral bending [48]. However the condition of the disks of these patients remains unknown and requires long-term clinical assessment.
Other designs allow more motion through the disk while providing selective force application required for spinal growth modulation [49]. The staple hemiepiphysiodesis technique consistently created spinal deformities in the coronal plane in a porcine model [26]. Driscoll et al. has reported using a hemi-staple which bridges vertebral body growth plates in a porcine model with the unique attribute that the staple does not cross a disk [50]. This model achieved growth modulation, evident by histological changes in the physes, and the disk remained viable; however, it is not certain how this technology may be applied clinically as there is no epiphysis in the human vertebral body.
In a study by Braun et al. [51], a variety of fusionless scoliosis implant strategies were tested in the rat tail model. This study used both rigid and flexible implants to modulate vertebral body growth. The results from that study demonstrated that dynamic loading of the vertebrae provided the greatest growth modulation potential. Aronsson et al. similarly showed that alternating compression and distraction applied to adjacent vertebrae in the calf tail could modulate vertebral growth, suggesting that dynamic motion would be preferred [52].
44.2.2 Basic Science Research Overview
Anterior vertebral body tethering provides an alternate approach for spinal growth modulation. Similar in theory to vertebral staples, the tether creates a compressive load on the anterior vertebral body and, through the Hueter–Volkmann principal, attempts to correct the asymmetric anterior spinal overgrowth [7–9, 53]. A theoretical advantage over vertebral staples, however, is that anterior tethering provides a less rigid construct in the directions of motion other than lateral bending away from the device and thus be less detrimental to long-term intervertebral disk health and spinal motion.
Anterolateral spinal tethering has been tested previously in various animal models. In 2002, Newton et al. [20] evaluated the effects of flexible mechanical tethering of a single motion segment. Eight immature calves were instrumented with anterior vertebral body screws over four consecutive thoracic vertebrae. Two screws were connected by a stainless steel tether, and two were left unconnected. After 12 weeks of growth, coronal and sagittal plane deformities were consistently created over the tethered motion segments, compared to control segments. In addition, vertebral body wedging was observed, indicating that physeal growth had likely decreased on the side of the tether. Biomechanical analyses revealed that the tether restricted lateral bending range of motion; however, this motion was found to return to control levels when the tether was removed. A follow-up study evaluating multilevel growth modulation in the bovine model concluded that given the adequate bony fixation, the flexible tether was able to consistently create a biplanar spinal deformity without having a detrimental effect on spinal motion [21]. Another study used an ultrahigh molecular weight polyethylene (UHMWPE) anterolateral spinal tether in a mini-pig model which more closely matched the size and growth rate of an adolescent patient. This study demonstrated the creation of vertebral body wedging in a tethered group of 14° after 6 months and 30° after 12 months, compared to a surgical sham group with no deformity creation (Fig. 44.3a, b) [25]. Additionally, anterior vertebral tethering of experimental scoliosis has been shown to alter growth, thus spinal contour and vertebral body shape, in three dimensions, decreasing coronal curvature, increasing kyphosis, and decreasing axial rotation [54].
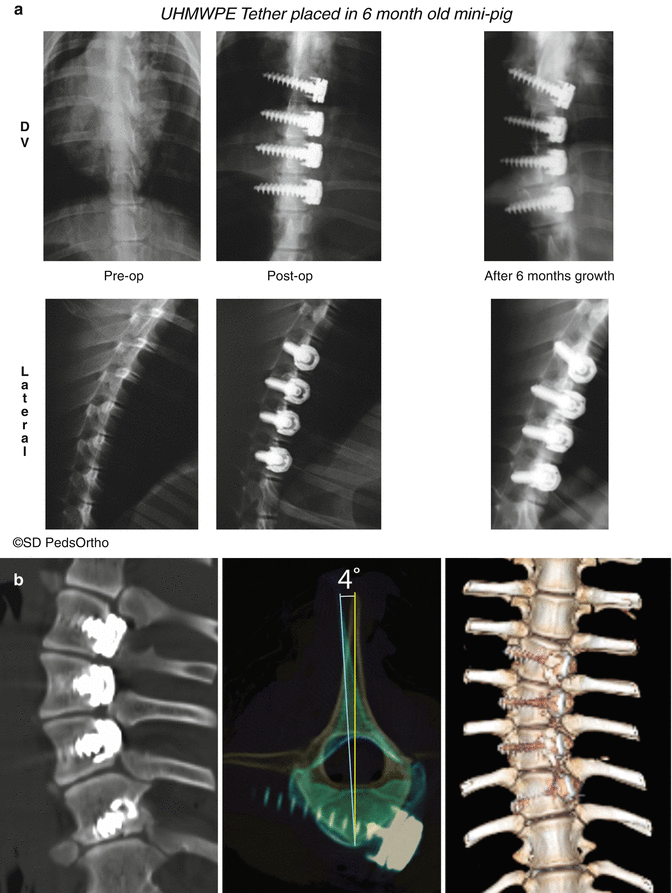
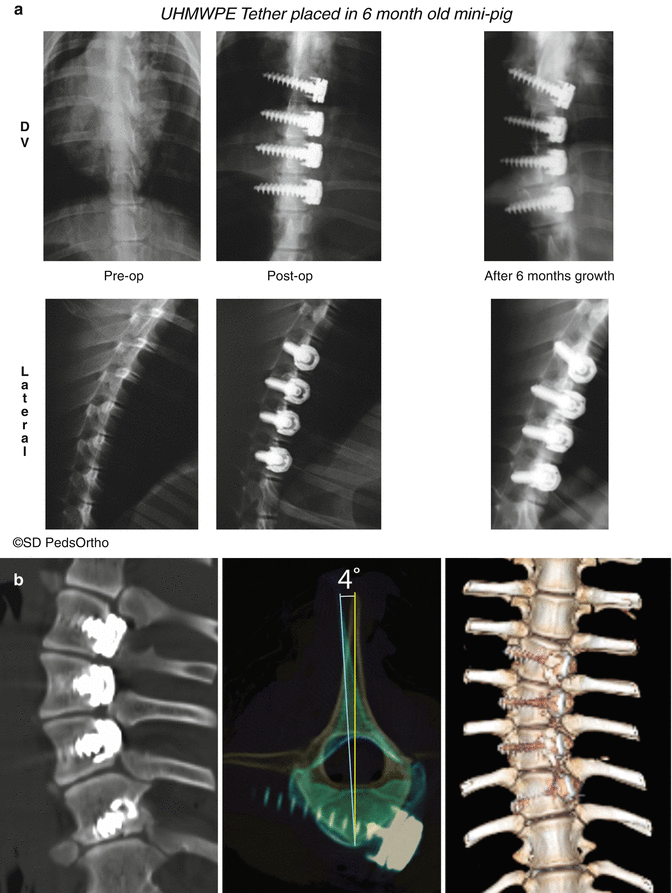
Fig. 44.3
(a) Radiographs showing creation of spinal curvature with a tether (radiolucent) in six months of treatment. (b) Representative computed tomography (CT) imaging done after 12 months of spinal growth modulation with a tether in a mini-pig. Midcoronal image demonstrates vertebral body wedging, axial image shows rotational deformity created, and anterior–posterior 3D CT image shows global deformity creation
In 2005, Braun et al. [17] compared the ability of shape memory alloy staples and bone anchor ligament tethers to correct an experimental scoliosis in 24 Spanish Cross-X goats. The flexible ligament tethers were found to improve scoliosis from an average of 73.4–69.9°, while scoliosis actually progressed in the goats treated with staples from an average of 77.3–94.3°. Pullout testing demonstrated that the bone anchors had improved integration into the vertebral bodies, while the staples were found to loosen. Histologic evidence of a halo of fibrous tissue around the staple tines was also presented and was thought to be responsible for staple loosening.
Intervertebral disk health has also been assessed after spinal growth modulation with anterior vertebral body tethers, as fusionless treatment strategies need to preserve the intervertebral disk if they are to be successful in the long term. Histologic and biochemical evaluation of intervertebral disk health after spinal growth modulation was reported by Newton et al. [22] Intervertebral disks from seventeen bovine instrumented with a multilevel flexible steel cable were compared with disks from 19 bovine that underwent a control sham surgery (screw-only). A double screw-double tether construct was required in this rapidly growing model to achieve adequate bony fixation. No change in disk water content or gross morphological grading was observed between the two groups; however, decreased disk thickness, increased proteoglycan synthesis, and a change in collagen distribution were present in the tethered disks. 3D disk reconstructions showed that the tethered disks were significantly shorter than sham disks (24 % decreased on left and 34 % decreased on right) [55]. Disk narrowing and wedging is seen in clinical scoliosis and in animal models which mimic the clinical situation [56]. In the mini-pig model, the vertebral body wedging created (convex on the side of the tether) was accompanied by wedging in the disks opposite to that in the vertebrae (convex on the contralateral side of the tether). Disks from spines with tethers were found to be hydrated, with MR signals similar to sham surgical spines (Fig. 44.4a–c) [23, 25]. Another concern is how the disk would respond to a tether with an applied tension, as would be the case in a clinical surgery where the surgeon desired at least a partial deformity correction intraoperatively. Previous work comparing tensioned and untensioned anterolateral spinal tethers in a mini-pig model was done using a thoracotomy and a tensioning device [24]. Results showed that tensioning to 250 N caused 8° of deformity over four vertebral levels, between three disks. The tensioned group did develop a deformity more quickly initially, but after 7 months of tethered growth, the deformities of tensioned and not tensioned were the same. Pretensioning the tether did not result in disk injury or a decreased ability for the growth to be modulated over time. Fusionless scoliosis implants have been found to result in alterations in intervertebral disk cell density [57] and collagen content; [22] however, the clinical implications of these changes are unknown. Further studies of disk health with noninvasive imaging modalities for the detection of early degeneration and after long-term growth modulation are required.
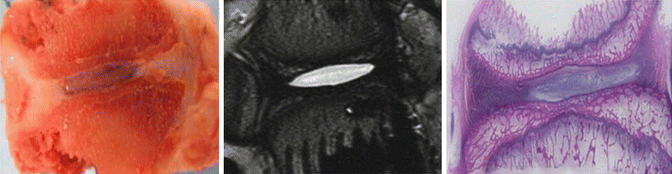
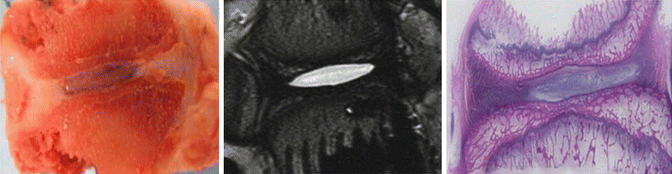
Fig. 44.4
Midcoronal views of intervertebral disks following tethering in a mini-pig model. Gross morphology demonstrates Thompson Grade 1 [83], T2 MRI demonstrates well-hydrated nucleus and intact annulus, and H&E-stained histology section shows all structures to be intact
The mechanism by which application of compressive forces unilaterally via vertebral tethering acts to modulate that the growth of vertebral bodies and of the global spinal contour is being explored and is just starting to be understood. Unilateral compression of a growth plate may result in changes to the growth plate. Indeed, Chay et al. found that tethering an experimentally created scoliotic curve in pigs resulted in a decrease in proliferative zone height on the side of the tether compared to the contralateral side of the vertebrae [58]. Others have found a decrease in hypertrophic zone height and cell numbers with a unilateral application of compression on the vertebral body [59]. Using 3D measurements of the growth plates from calves that had tethers placed, Newton et al. likewise showed a thinning of the physis on the side of the tether in the tethered spines [55]. In all of these studies, even though the growth plates were altered from their normal state, they remained open for the duration of the treatment, and thus potential future growth was preserved. Further work in animal models involving growth plate response to changes in the mechanical environment continues and is particularly promising as growth cartilage in various bones across various species seem to respond similarly; thus, it could be especially appropriate to apply to growth in human vertebrae [60].
44.2.3 Posterior Spinal Tether
Vertebral tethers have also been used in the posterior spine to modulate spinal growth. In 2005, Lowe et al. [61] evaluated the ability of polyethylene cords to effect sagittal alignment in an immature nonfusion segmental pedicle screw sheep model. At 13 months after surgery, the tethered animals had significantly less kyphosis and vertebral body wedging than the control animals. The authors concluded that the posterior tether may be a potential treatment for adolescents with Scheuermann’s disease. However, there are no clinical reports of use of a tether to correct this deformity, and the usefulness to modulate growth in Scheuermann’s disease, which often presents after the adolescent growth spurt [62], is yet to be studied.
44.3 Anterolateral Spinal Tether: Clinical Application
44.3.1 Indications for Intervention
The specific indications and contraindications for juvenile spinal growth modulation are evolving with greater clinical experience. The ideal application is likely for the treatment of preadolescent idiopathic scoliosis with >40° curves that have a high likelihood of progression. However, it may be the best treatment choice in curves with higher magnitudes and in those that are progressing beyond the range that is considered to be braceable (>45°). Recently, it has been suggested that tethering may become the growth-modulating technique of choice for curves greater than 40° that are beyond the treatment range for vertebral body stapling effectiveness [63]. Our current indications to consider tethering are for patients with primary thoracic curves of 45–65° in females who are Risser 0 and males Risser 0 or 1. The thoracic spine should be hypokyphotic. Indications for tethering thoracolumbar curves remain unclear. It is very important that patients are carefully screened and their family situation is well known to be stable so that they remain available for close monitoring – as with all growth-modulating applications, there is a risk of overcorrection and the consequences can be serious.
44.3.2 Timing of Surgery
The timing of tether placement is crucial to its success. Treatment needs to be initiated in time to allow enough growth to modulate the vertebrae to become “straight.” However, if the tether is applied too early, the risk of over correction increases. At this point, there are no clinical studies, as there are for lower limb length inequality [64, 65] that allow for definitive determination of what age/skeletal maturity stage is ideal, not too early or too late, to intervene. The determination of the amount of remaining growth in a candidate patient is essential and has been traditionally determined using the Greulich and Pyle method which uses a hand x-ray to determine skeletal age based on an atlas of skeletal development published in 1959 [66]. In routine clinical practice, the Risser grade of ossification of the ilium (grade 0–5) has been routinely used to determine skeletal maturity, and only children with Risser of 0 or 1 have been considered young enough to benefit for a spinal growth modulation procedure. The Risser sign remains 0 (no ossification of the iliac crest) until after a patient’s peak spinal growth velocity has passed. Sanders et al. have found that open triradiate cartilage is a strong predictor of a child being at their peak or pre-peak of spinal growth velocity which is associated with continued anterior column growth [67]. The triradiate cartilage closes prior to the appearance of a Risser sign and therefore is an earlier predictor of the beginning of maturity. As such, open triradiate cartilage may be the best current criteria for determining that a patient has “enough” remaining growth to maximize the tether effect for larger curves. The Tanner-Whitehouse-III RUS scores correlate skeletal age of the metacarpals and phalanges with scoliosis curve progression and can show maturity stages in the age range where triradiate cartilage is open; thus, it is an earlier predictor of the growth “spurt.” [68] One method of determining skeletal growth remaining and the likelihood of scoliosis progression that is more practical in a clinical setting is proposed by Sanders et al. and uses a modification of the Tanner-Whitehouse-III score [69]. Another popular method for assessing skeletal maturity, particularly for patients with open triradiate cartilage, is the Sauvegrain method which correlates elbow ossification centers with peak skeletal growth [70, 71].
44.3.3 Intraoperative Considerations
In the 1990s, the use of the thoracoscopic approach to the spine [72] became popular as a safe [73] means for anterior thoracic discectomy to provide spinal flexibility for improved deformity correction [74–76] and to allow anterior fusion to prevent crankshaft deformities [77, 78]. Anterior instrumentation has also been successfully placed in main thoracic scoliosis, with 5-year postoperative outcomes comparable to those achieved for open anterior and posterior techniques. [79] Two decades later, the widespread safe use of pedicle screws as a powerful means for deformity correction and stabilization greatly decreased the need for anterior discectomies, and thus, the use of the thoracoscopic approach also decreased; however, it is still used for very severe and stiff curves and other select cases with specific indications for anterior release and/or fusion [80]. The thoracoscopic approach is experiencing recent renewal with anterolateral growth modulation techniques, including the tether, as new applications of the approach.
The current experience using anterolateral tethering techniques is discussed here; however, this technique remains novel, and there is very little published experience in its clinical application and no published reports on the clinical use or outcomes of the technique.
44.3.4 Thoracoscopic Approach
The authors prefer a thoracoscopic approach for tether insertion. Basic thoracoscopy skills are required in order to safely navigate the chest cavity (Fig 44.5a–c). Spinal deformity surgeons may find the skills of a general/thoracic surgeon with thoracoscopic experience a great value in performing this procedure. The details of performing thoracoscopic spinal instrumentation can be found in other sources [81, 82]. Briefly, the lungs are selectively ventilated allowing the lung on the convex side to collapse creating the working space. As with traditional instrumentation for fusion, three 15 mm ports are placed along the posterior axillary line in positions that will allow direct lateral access for screw placement. A single anterior axillary line portal at the apex of the curve is used for scope placement (See Fig. 44.6a). The pleura is incised longitudinally, and the segmental vessels are coagulated and divided using ultrasonic energy. The azygous vein and great vessels are dissected from the anterior spine by packing a sponge between these structures and the spine. This is an added step that creates space and protection when the vertebrae are drilled, tapped, and screws placed.
