Fig. 45.1
(a) Radiographs from preclinical trial of first implant design, knee hemiepiphyseal staples with shortened tines. Left: Immediate postoperative. Right: 6 weeks postoperative. Bone fixation was obviously insufficient, but local curvature is suggestive at the center, stable, implant level. (b) Radiographs from preclinical trial of second implant design, custom stainless steel implant with one bone screw per implant. Left: Immediate postoperative. Right: 2 months postoperative
To help quantify the Hueter-Volkmann principle, the relationship between physis function and mechanical stress, a study on a readily available clinical model of growth modification was performed [8]. Knee stapling in adolescents with genu valgum was analyzed (Fig. 45.2) under the assumption that the stress, i.e., force divided by cross-sectional area, that a growth plate can exert has a constant maximum value. The study showed that the force to correct knee angular deformities in adolescents was approximately 500 N, or slightly more than the normal body weight, which implied that a compressive stress of about 1 MPa is needed to stop the growth. At the same time, a study in scoliosis patients showed that vertebral body physes continue to exist even on the concave side of the apex of large curves in older adolescents [9] (Fig. 45.3).
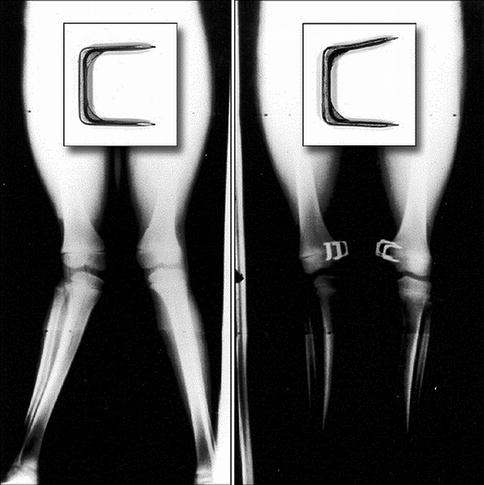
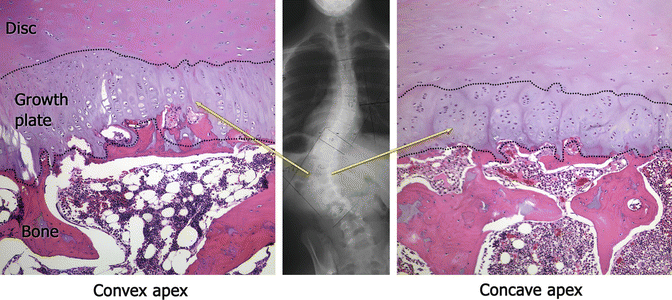
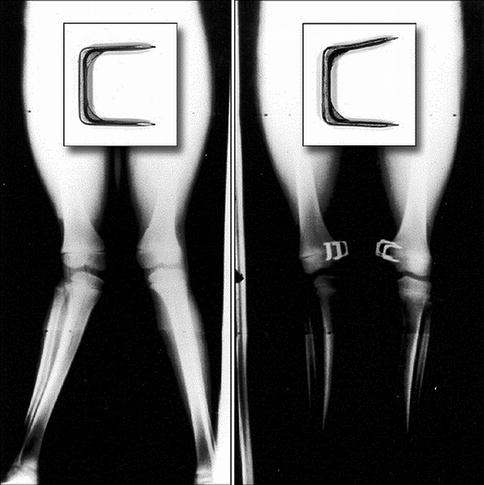
Fig. 45.2
Forces and stresses of growth were determined by experimentally reproducing deformations of hemiepiphyseal staples measured from serial radiographs of adolescents treated for genu valgum
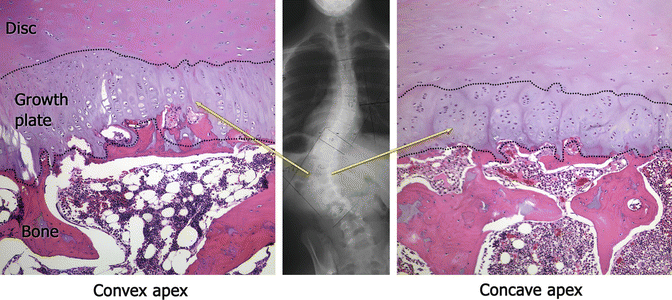
Fig. 45.3
Radiograph and histological sections of vertebral physis from scoliosis patient, 12-year-old male, 74° curve. Arrows indicate the side and level of curve sections. Dotted lines outline the physis. On the convex side both the hypertrophic cells and the hypertrophic zone of the growth plate are larger than those on the concave side
Repeatable results were shown using the porcine inverse analog model, with curvatures increasing with postoperative time [10]. In order to determine if the mechanism of increasing curvature included changes to the vertebral physes, rather than consisting solely of asymmetric disc compression, a histomorphometric study was performed [11]. The study showed decreases in the size of the physeal structures which correlate strongly with growth rate, the height of the hypertrophic zone, and hypertrophic cell size on the treated side [12]. These side-to-side differences in growth (Fig. 45.4) indicated asymmetric vertebral growth. Related biomechanical, mechanobiological, and computational [13] studies helped determine initial displacements [14], stresses [15–17], and ranges of motion [18].
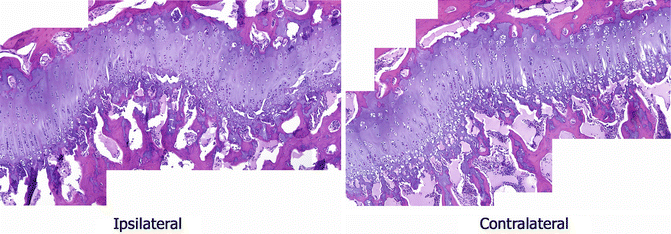
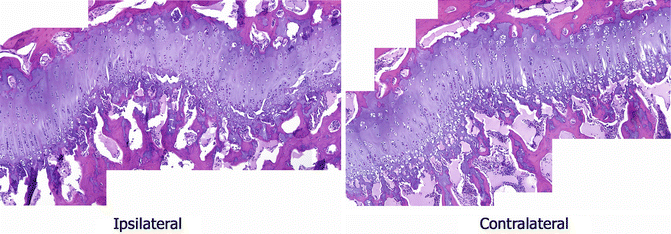
Fig. 45.4
Histological sections of vertebral physis from skeletally immature domestic pig from preclinical trial of last prototype implant of stainless steel with two vertebral body screws. Curvatures were induced in five consecutive animals. Left: Sample from treated side, within implant tines. Right: Sample from the opposite side of the same level. Qualitatively, the images suggested changes to the growth plate and bone at the instrumented level on the side ipsilateral to the implant. Quantitative structural differences were reported [11]. Similar to the human in Fig. 45.3, the hypertrophic cell height and the hypertrophic zone height of the stapled animals were greater on the side of the vertebrae contralateral (opposite) to the staple versus the side ipsilateral to the staple
In preparation for human use, including implant design changes and titanium rather than the stainless steel implants, several preclinical evaluations were conducted. To align with procedural requirements of the US FDA, in vivo tests were conducted in an independent contract with Good Laboratory Practices (GLP) facility [19]. Curves again increased significantly. Results corroborated the repeatability of curve induction in this model (Fig. 45.5). The combined studies demonstrated that the system was effective in inducing asymmetric growth modulation in a quadruped model. Additional conclusions were that the clips and vertebral locking screws were able to be explanted, that the clip and screws remained secure in vivo, and that the clips showed no evidence of permanent deformation.
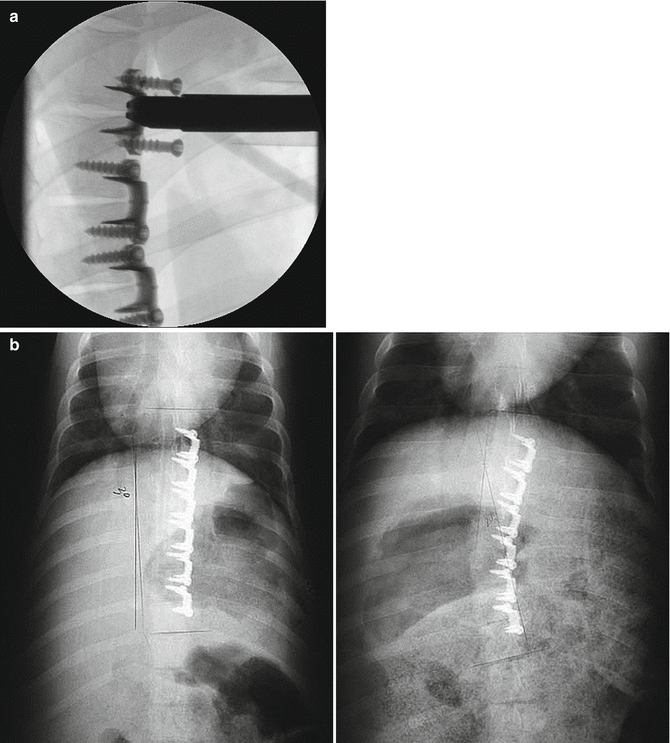
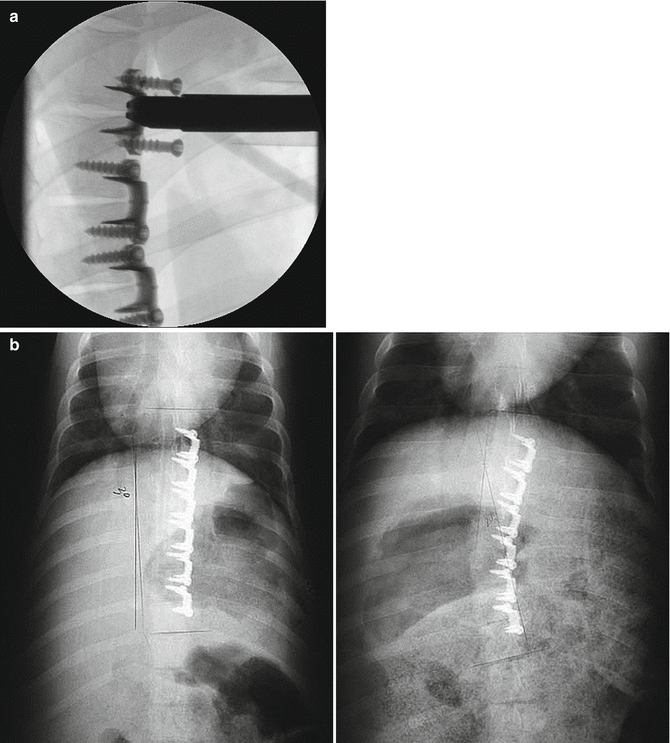
Fig. 45.5
Radiographs from preclinical in vivo study of titanium clip-screw construct in porcine model. (a) Intraoperative fluoroscopic image showing clip with two preloaded screws in insertion tool. (b) Left: Immediate postoperative curve is 3°. Right: At 8 weeks postoperatively, the major curve is 20°
45.3 Clinical Study
45.3.1 Objectives and Design
A prospective, FDA Investigational Device Exemption (IDE), clinical safety trial was then initiated [20]. The novel intervertebral titanium clip device and surgical instrumentation were designed for the treatment of progressive idiopathic scoliosis (IS) by modulating spinal growth (hemiepiphysiodesis) without the need for spinal fusion. The device was designed as a fusionless, growth modulation system to provide an alternative to external bracing especially for patients who have a lower chance of success with brace wear [21–23]. The system is intended for patients who are diagnosed in the early stages of IS and have a high potential for curve progression.
The mechanical objectives of the clip/screw device are to provide compression on the convex side of the curve and mild distraction on the concave side. The implants are intended to redirect asymmetric growth by distributing vertebral growth-altering stresses on the main thoracic spine segment and by promoting the secondary curves to align and balance. Insertion of the implants realigns the vertebrae, as may be noted in the side-to-side change in disc height in the intraoperative fluoroscopic images (Fig. 45.6) and in the immediate postoperative thoracic curve reductions in the first standing radiographs. This slows growth on the convex side of the curve and may enhance growth or decompress the disc on the concave side. The mechanical design criteria incorporate evidence of the forces of growth and growth modulation stress gradients, as well as physiologic ranges of motion and loading. The construct consists of a clip and two vertebral locking screws (Fig. 45.7) which are placed on the lateral aspect of the thoracic and thoracolumbar spine. The device is designed and sized to bridge superior and inferior physes and intervening disc.
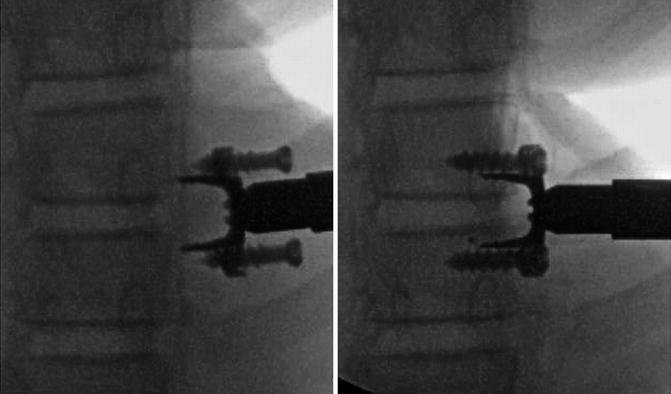
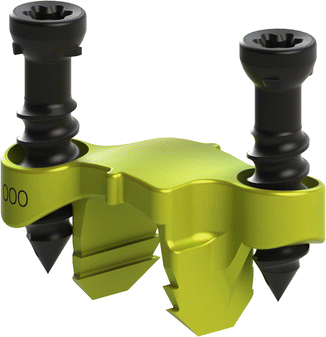
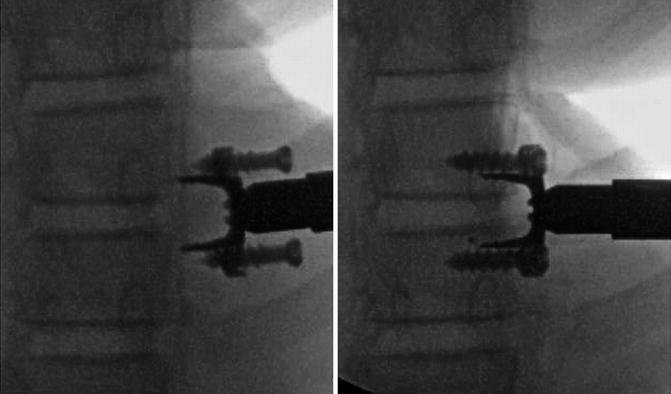
Fig. 45.6
Intraoperative fluoroscopic images from clinical trial show titanium clip with preloaded screws in insertion tool before (left) and after implantation (right). The disc is slightly compressed on the implant side and distracted on the opposite side
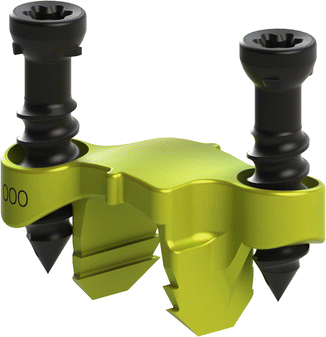
Fig. 45.7
Titanium clip-screw implant construct is shown with preloaded screws
Like staples in long bones, evidence indicates that the device inhibits growth asymmetrically. The construct may be viewed as a combination of a knee hemiepiphyseal staple and an 8-plate. As vertebral growth gradually increases, the time-averaged compressive stress on the physis also increases, which slows growth on the side ipsilateral to the implants. If the average relative compression on the concave side is lowered, the method may also allow for a growth rate closer to normal at the concavity. Disc motion is partially retained. Postoperatively, the compressive stress on the disc and the physis within the tines increases [17] and reaches a side-to-side gradient of a magnitude sufficient to inhibit growth asymmetrically, that is, a time-averaged spatial stress difference of between 0.1 and 1 MPa [8, 24]. Although scoliosis involves growth disturbances in three dimensions, it appears possible that if the thoracic coronal curvature is modified early and sufficiently, and if the initial curve is not too stiff or axially rotated, the otherwise inexorable progression of compensatory curves, rib deformities, and axial rotation may be interrupted or perhaps even corrected.
45.3.2 Therapeutic Goals and Theoretical Advantages
Titanium clip-screw implant constructs:
Arrest or correct idiopathic spinal deformity without fusion or long-term bracing.
Maintain quality of life; impose few activity restrictions.
Eliminate compliance with brace dosing schedules.
Avoid fusion, allow for continued growth of the thorax, and increase chest volume for pulmonary function.
Apply forces at the vertebral body physes, as opposed to external braces that apply forces to the spine indirectly.
Spare motion and disc height, which may allow for sufficient disc health maintenance.
Allow for fusion instrumentation later if necessary.
Use advantageous material and structural properties of titanium: biocompatibility, rarity of allergic reactions, flexibility in bending, resistance to fatigue, and osseointegration.
Allow for entirely thoracoscopic approach and implantation.
Provide for reversible procedures for implant removal with thoracoscopic procedures
Reduce operative time and blood loss compared to fusion and so avoid transfusion and keep anesthesia time low.
Substantially reduce rehabilitation and recovery time compared to fusion.
Provide strong safety profile: relatively small implants, no bi-cortical instrumentation, no risk to opposite side vessels, very low risk of spinal canal penetration, and low risk of disc encroachment during or after procedure.
Ease surgical procedures compared to other types of growth modification systems: few parts, no large vertebral body screws, fewer possible variations in implant procedures, and no material phase change to induce implant deformation for shape change.
Deliver relatively low dose of mechanical compression force and so low probability of overcorrection requiring reoperation. Largely limit biomechanical effects to individual motion segments: no direct interconnection between devices and no end-to-end ties so moments acting on implant fixation sites and laterally directed pull-out forces exerted on screws remain low compared to systems that connect several motion segments.
45.3.3 Patient Selection Criteria for Early Clinical Trial
The primary patient selection criteria for the pilot clinical trial in humans are summarized in Table 45.1
Table 45.1
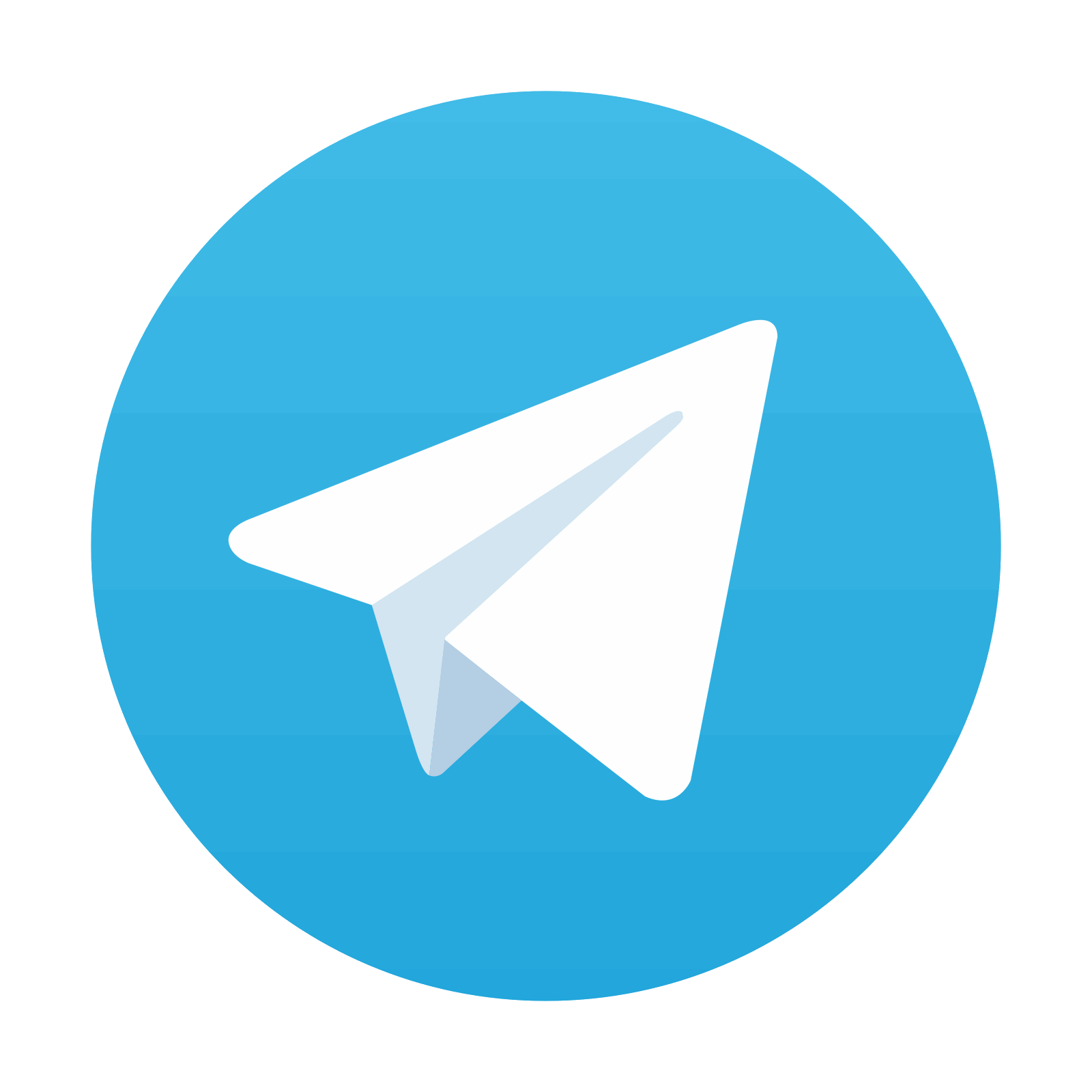
Patient selection criteria for early clinical trial
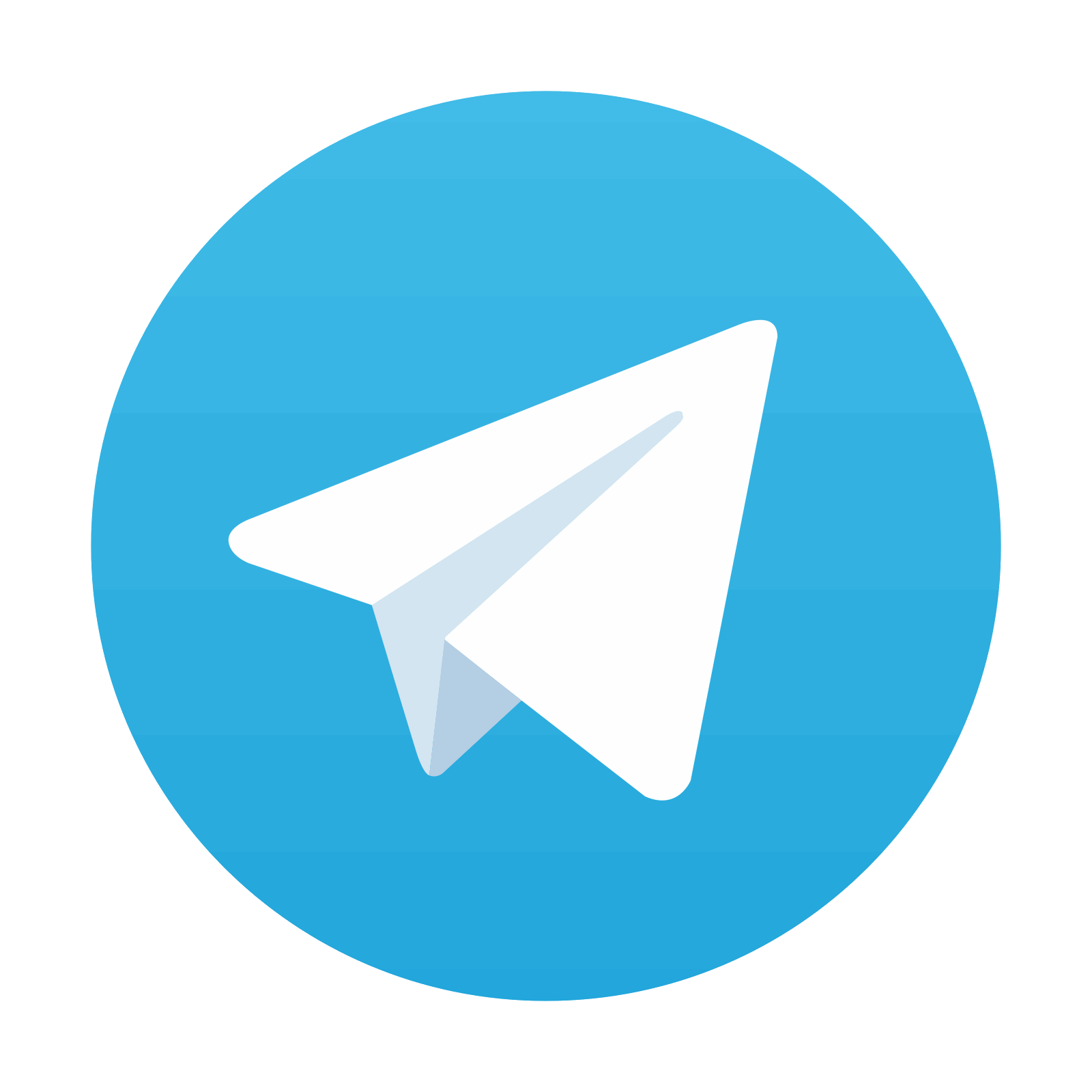
Stay updated, free articles. Join our Telegram channel

Full access? Get Clinical Tree
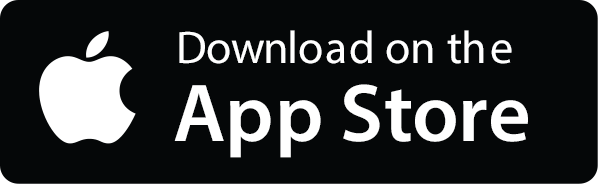
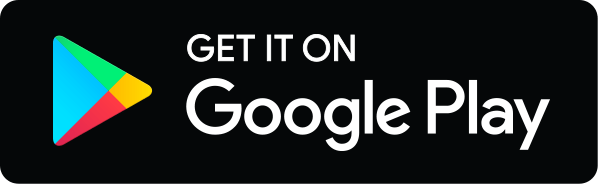
