Fig. 53.1
Electrode positions for posterior tibial nerve SSEP, H-reflex and train of four (TOF). (TOF is monitored to ensure clearance of the neuromuscular junction for optimal tceMEP recordings.) (1) Left, popliteal fossa (H-reflex stimulation site); (2) right, popliteal fossa (H-reflex stimulation site); (3) left, gastrocnemius muscle (H-reflex and tceMEP recording site); (4) right, gastrocnemius muscle (H-reflex and tceMEP recording site); (5) right, posterior tibial nerve (TOF and SSEP stimulation site); (6) right, abductor hallucis muscle (TOF and tceMEP recording site). The left posterior tibial nerve stimulation electrodes are not shown
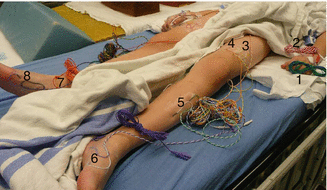
Fig. 53.2
Electrode position for recording upper and lower extremity tceMEPs. (1, 2) Left, first dorsal interosseous muscle; (3, 4) left, quadriceps muscle; (5) left, tibialis anterior muscle; (6) left, abductor hallucis muscle (also used for TOF recording); (7) right, posterior tibial nerve SSEP and TOF stimulating site; (8) right, abductor hallucis muscle (also used for TOF recording)
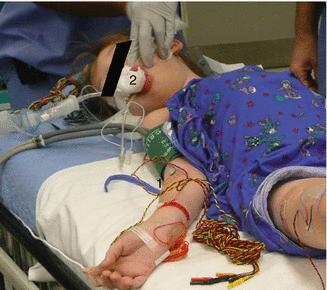
Fig. 53.3
(1) Recording electrode over the right deltoid and biceps muscles for upper extremity tceMEP recordings during VEPTR procedure. (2) Position of the bite block for tongue bite protection
53.3 Neuromonitoring Modalities
Despite the early historical success of SSEP monitoring during surgical correction of scoliosis, reliance on this modality is no longer adequate for the growing population of patients who present for surgical correction of increasingly complex deformities. As the SSEP is mediated by the posterior sensory columns and reflects integrity of spinal cord white matter, it provides no direct information about the condition of the descending motor tracts or spinal cord gray matter structures which are particularly susceptible to vascular insult. Hence, when used alone to monitor spinal cord function, SSEPs carry a definite risk of false-negative findings, even among patients with adolescent idiopathic scoliosis [1, 3, 4]. Because of the distractive or lengthening maneuvers needed for deformity correction both with traditional fusion and contemporary nonfusion techniques, there is increased opportunity for excessive vascular stretch and ischemic spinal cord injury. This may not manifest in the SSEP, either at all, or within the critical period necessary to initiate timely intervention for injury reversal.
In response to the limitations of SSEP monitoring, other techniques have been introduced to assess the descending spinal cord motor tracts and anterior horn function. These include transcranial electric motor-evoked potentials (tceMEPs) and the Hoffmann reflex (H-reflex). The highly debated and often misunderstood neurogenic “motor”-evoked potential (NMEP) is discussed separately in this chapter because of several seminal studies that point to its sensory origin.
53.3.1 Transcranial Electric Motor-Evoked Potentials
tceMEPs are neuroelectric events elicited from descending motor pathway structures including the corticospinal tract (CST), spinal cord interneurons, anterior horn cells, peripheral nerves, and skeletal muscles. These potentials are triggered by delivering electric pulse trains to the brain through subdermal scalp electrodes over the motor cortex, as illustrated in Fig. 53.4. Following depolarization of the cortical motor neurons, efferent neural signals course through the internal capsule to the caudal medulla where CST fibers decussate and descend into the spinal cord motor tracts. CST axons enter the spinal cord gray matter, interact with spinal interneurons, and go on to synapse with alpha motor neurons that innervate peripheral muscle.
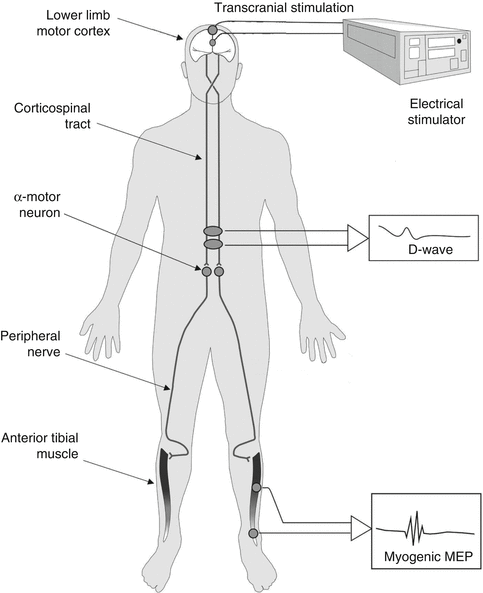
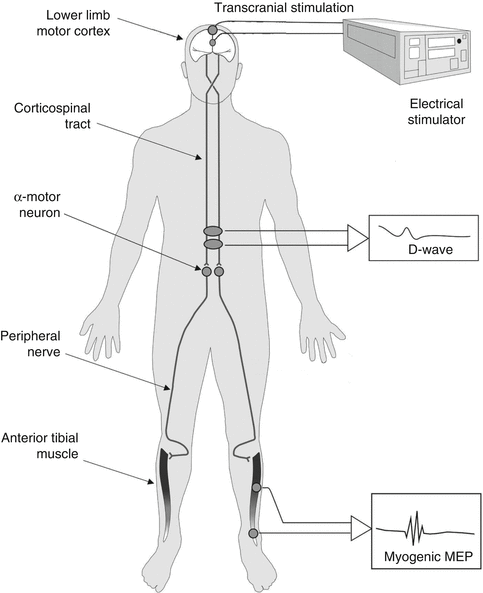
Fig. 53.4
Schematic of tceMEP stimulation and recording
Compound muscle action potentials representing motor-evoked potentials can thus be recorded from upper and lower extremity peripheral muscle with subdermal needle electrodes at the end of this neural chain. Because of the high sensitivity–specificity of tceMEPs for the identification of spinal cord and spinal nerve root injury [3–7], they should now be considered as the gold standard for monitoring spinal cord motor function during complex spine surgery.
The sensitivity of tceMEPs to motor pathway insult is illustrated in Fig. 53.5 which shows the time course of tceMEP monitoring in a 9-year-old female undergoing revision of growing rod for the treatment of neuromuscular scoliosis. This child presented preoperatively with bilateral upper and lower extremity weakness, though she was weight-bearing and capable of taking several steps with support. Reference to Fig. 53.5 shows that soon after placement of pedicle screws at T2–3, there was acute tceMEP amplitude diminution at left tibialis anterior (TA) and right abductor hallucis (AH) recording sites and complete loss of the right tibialis anterior muscle response. Moments later, the patient became hypotensive with a mean arterial pressure (39 mmHg) well below the threshold level for spinal cord ischemia (see Chap. 51 for further discussion). At this time, there was bilateral loss of lower extremity tceMEPs, with the exception of a barely observable response (10 % of baseline amplitude) from the left AH muscle. Note, however, that control responses from the left and right upper extremities (first dorsal interosseous muscle) remained unchanged.
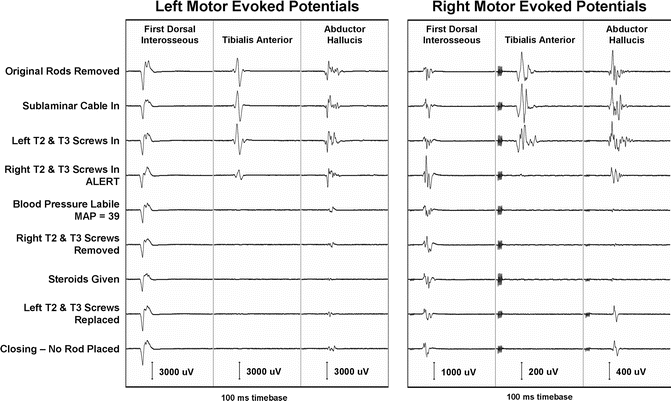
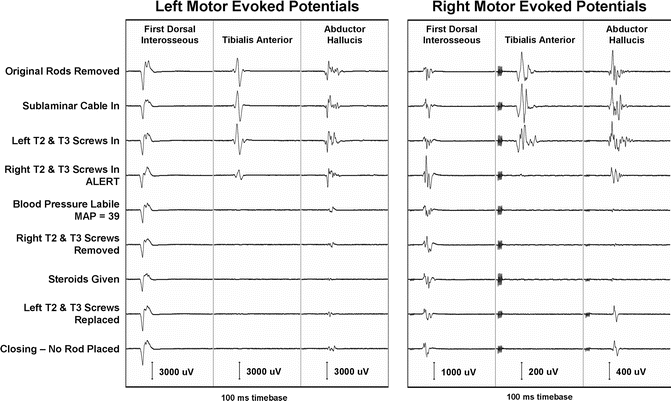
Fig. 53.5
Time course showing acute tceMEP loss following pedicle screw placement at T2–3 in a 9-year-old female undergoing revision of growing rod for treatment of neuromuscular scoliosis
Despite all attempts to raise the blood pressure, replace screws, and begin a spinal cord injury course of methylprednisolone, the tceMEP amplitudes never improved. Predictably, the child emerged from anesthesia exhibiting further deterioration of lower extremity motor function.
53.3.2 The Hoffman Reflex
The H-reflex, recorded from gastrocnemius muscle following stimulation of the tibial nerve at the popliteal fossa, is a neurophysiological correlate of the ankle stretch reflex influenced by upper motor neurons and other supra-segmental structures of the spinal cord and brain. The H-reflex reflects the functional integrity of complex motor control subsystems in the highly integrated ascending, descending, and interneuronal pathways of the spinal cord. It is thought that severe, acute spinal cord injury results in suppression of the H-reflex, which is observable within moments of insult. When amplitude suppression has exceeded 90 % and has persisted for the rest of the surgery, patients have awakened with profound postoperative neurological deficit consistent with spinal shock [8].
Because of its neurophysiological underpinnings and the fact that the anesthesia requirements for recording an H-reflex are not as restrictive as those for tceMEP monitoring, a minority of clinical neurophysiologists have proposed H-reflex monitoring as an equivalent substitute for tceMEPs [9]. Hicks [10] compared the results of H-reflex monitoring to the postanesthesia ankle clonus test in a diverse population of 292 spine surgery patients. The H-reflex predicted the outcome of the ankle clonus test in 80 % of the cases, leading Hicks [10] to conclude that the H-reflex was a reliable predictor of spinal cord injury during spine surgery. Of the three patients in the series who exhibited loss of the H-reflex intraoperatively, all had subsequent recovery to baseline prior to closure. There were no patients with permanent loss of the H-reflex and none with postoperative deficits, making comparison of results with known sensitivity of tceMEPs to spinal cord injury difficult at best.
Our experience with the H-reflex has not been as positive as that reported by others, particularly in very young children. All too often, the responses are either variable or there is significant inter-leg amplitude asymmetry for recorded H-waves. Moreover, we have observed dissociations between H-reflex responses and tceMEPs on several occasions, suggesting that the former may be less sensitive than the latter to predisposing factors for spinal cord injury.
An example of such dissociation is illustrated in Fig. 53.6. This figure shows intraoperative transcranial electric motor-evoked potentials and H-reflex responses recorded from a 42-month-old female during a third lengthening of growing rod to treat a 68° kyphotic deformity of the thoracic spine. The patient’s history is significant for the resection of thoracic teratoma at 1 month of age, initial placement of growing rod at 10 months with removal at 15 months, and subsequent biopsy of spinal cord lesion with reinsertion of growing rod at 23 months of age.
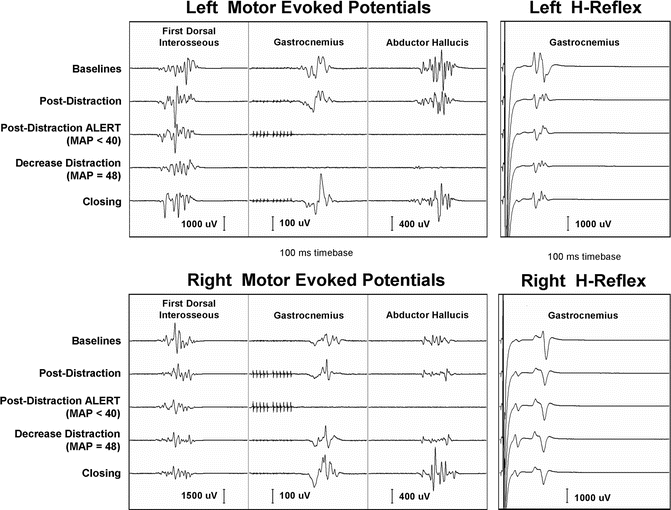
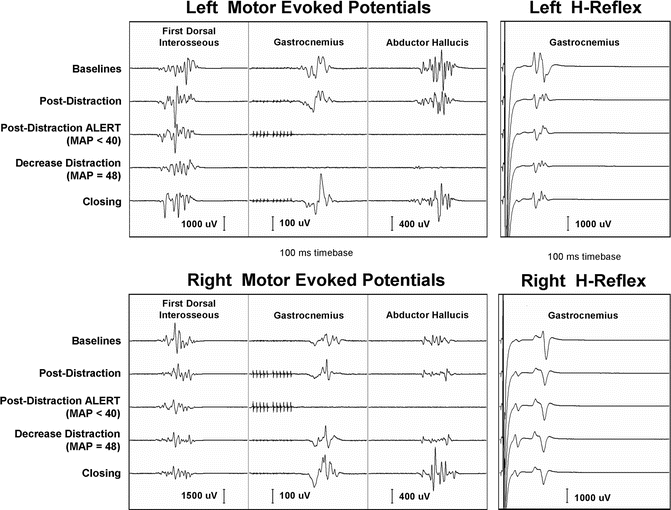
Fig. 53.6
Example of the dissociation between transcranial electric motor-evoked potentials and H-responses recorded from a 42-month-old female during a third lengthening of growing rod. Note the loss of tceMEPs with no significant change in H-responses
Following lengthening of the growing rod, tceMEPs disappeared from multiple lower extremity myotomes, including bilateral tibialis anterior, gastrocnemius, and abductor hallucis muscles. There were no concomitant changes in the H-waves recorded from bilateral gastrocnemius muscles during this period. Upon decrease in distraction and elevation of mean arterial blood pressure, motor-evoked potential amplitudes returned to baseline range. There were no new postoperative neurological sequelae. In light of such examples, where H-reflex responses have been insensitive to surgical and physiological conditions known to be risk factors for postoperative deficit, we have come to view its role in neuromonitoring somewhat differently from that described by Hicks [10] or Toleikis and Toleikis [9].
Unlike tceMEPs which are both highly sensitive and specific for evolving spinal cord injury, the H-reflex appears to be more specific than being sensitive. That is, while presence of the H-wave cannot be equated to a prediction of no neurological injury, acute loss of the response could point to spinal shock. For that reason, we would caution not to view the H-reflex as a substitute for tceMEP monitoring, but rather as an adjunct. Moreover, in the presence of discrepancy between the results of the two monitoring modalities, greater interpretive weight should be given to tceMEP findings. To be sure, it is not a “new standard” as posited by Toleikis and Toleikis [9].
53.3.3 Neurogenic-Evoked Potentials are Sensory Not Motor
There is considerable evidence dating back to 1992 that the NMEP predominantly reflects the functional integrity of posterior column sensory tracts rather than lateral and anterior column motor pathways [11–18]. The general conclusion drawn from these studies is that the NMEP is triggered by antidromic activation of posterior column sensory fibers that communicate with alpha motor neurons via collateral branches at a segmental level. Thus, NMEPs are insensitive to a wide variety of surgical and vascular insults to the motor tracts of the spinal cord by virtue of the sensory pathways that mediate them. As such, it may be more appropriate to label these responses NSEPs (neurogenic sensory-evoked potentials) or DASEPs (descending antidromic sensory-evoked potentials).
Despite the body of evidence pointing to a sensory origin for the NMEP, it continues to have its proponents as a viable technique for monitoring the spinal cord [19, 20]. While there is a general consensus in the surgical neurophysiology community that its use in place of the tceMEP provides a false sense of protection for spinal cord motor tract function, the ultimate decision to continue monitoring with NMEPs lies with the neurophysiological monitoring personnel and surgeons at each hospital facility [21].
In our experience, neurogenic-evoked potentials have played a limited role as an adjunctive modality for monitoring spinal cord sensory function, since we began the routine use of tceMEPs to monitor the motor tracts over 15 years ago. The contribution of neurogenic-evoked potentials is further limited by observations that they can disappear in the presence of rigid spinal instrumentation due to current shunting [22]. This creates interpretative ambiguity for the neuromonitoring professional and can result in a false-positive result just at the time of deformity correction, leading to unnecessary intervention.
53.3.4 Monitoring Spinal Nerve Roots
To the extent that growing rods are anchored to the spine with pedicle screws, individual spinal nerve roots may be at risk of injury from medial pedicle breaches and should be monitored using spontaneous and electrically stimulated electromyography, as well as tceMEPs [7]. The principles and techniques for testing pedicle screw placement with electrical stimulation described in considerable detail for the mature spine by Schwartz et al. [7] are equally applicable to the growing spine. Note that stimulation threshold criteria for detection of medial pedicle breaches may have to be adjusted downwards from those reported for adults to account for smaller pedicles in children. An increase in the use of image-guided navigational placement of pedicle screws coupled with either O-arm or fluoroscopic post hoc analysis of screw placement has also gained popularity among spine surgeons. This technique allows the surgeon to visualize the complete path of the pedicle screws to rule out medial, lateral, or other potentially unsafe trajectories at a sensitivity equivalent to traditional pedicle screw testing [23]. In some centers, pedicle screw placement under O-arm navigation has supplanted stimulated EMG for detection of medial pedicle wall fracture from an improperly directed screw, while in others, stimulated EMG serves as an adjunctive test to the O-arm gold standard.
53.3.5 Monitoring the Brachial Plexus
There has been recent interest in the evaluation and treatment of patients who have a congenital spinal deformity together with chest-wall abnormalities leading to thoracic insufficiency syndrome. The VEPTR has been effectively utilized for the treatment of these challenging patients by expanding the chest during the growing years to allow for maximum lung development, while also treating the spinal deformity. As is common with a new technique, there is a debate on the value of intraoperative neuromonitoring during VEPTR surgery.
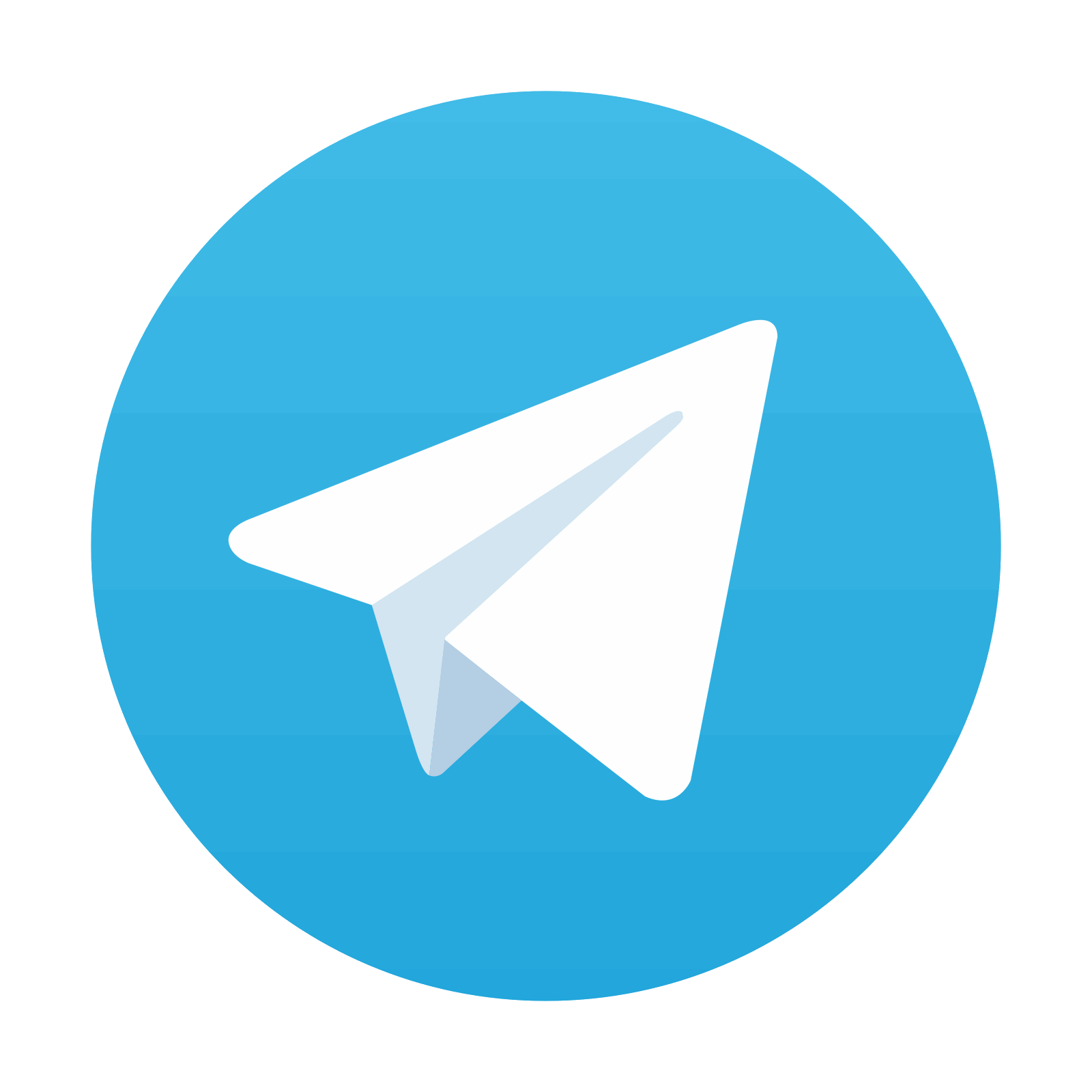
Stay updated, free articles. Join our Telegram channel

Full access? Get Clinical Tree
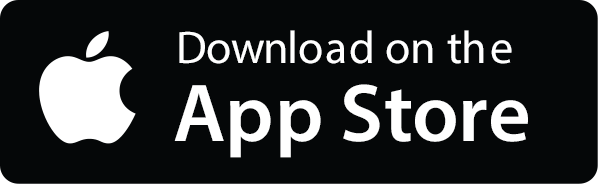
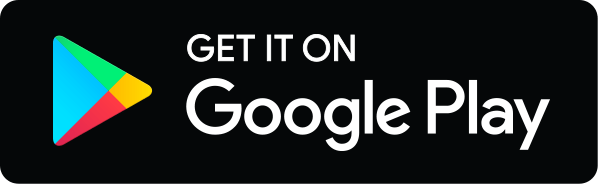