html xmlns=”http://www.w3.org/1999/xhtml” xmlns:mml=”http://www.w3.org/1998/Math/MathML” xmlns:epub=”http://www.idpf.org/2007/ops”>
While the neurological examination represents a time-honored gold standard for diagnosing and tracking the clinical course of disease, the sensitive and accurate assessment of central nervous system integrity remains a challenge. Optical coherence tomography (OCT) is an effective tool that has emerged over the past decade for measuring structure–function correlations and quantifying pathological changes invisible to the human eye. OCT can complement the clinical neurological examination in ways that are similar to magnetic resonance imaging (MRI), a tool that has given us tremendous structural insights into the nervous system. As we begin to understand the remarkable capacity of the brain to compensate for neurological injury, the links between structure, function, and electrophysiology that are provided uniquely by the afferent visual pathway will be critical. The relative timing of injury to the retinal ganglion cells (neurons), retinal nerve fiber layer (axons), and other ocular structures may be unique to each neurologic disorder. Such similarities outweigh differences, and the visual pathway represents an attractive global model for understanding and monitoring disease.
Neurologists acknowledge the capacity for noninvasive imaging technologies to accelerate and optimize neurologic diagnosis and follow-up. This is perhaps best illustrated by the pivotal role of MRI in the diagnosis and management of multiple sclerosis (MS). The field of neurology has witnessed a monumental transformation from near complete reliance on clinical history and examination to the addition of MRI and other noninvasive imaging techniques. An extension of the clinical examination, MRI has revolutionized care not only in MS but in the case of other neurologic disorders. For example, the relative specificity of MRI findings in some cases is helpful diagnostically. In this regard, OCT continues to evolve in its capacity to identify findings unique to any single neurologic or ocular disorder. While the architecture of the neural retina has been nearly totally inaccessible even with the application of the most sophisticated and high-precision MRI methods, the advent of high-resolution spectral-domain (SD) OCT has fundamentally changed our ability to qualitatively and quantitatively assess the eye – the most visible part of the human brain.
Neurological disorders frequently present with, or ultimately involve, visual impairment. Greater than 50% of the brain’s pathways are dedicated to functional networks related to the visual system. While ocular motility is germane to foveation and subsequent image processing, the determination of the location and underlying mechanisms that compromise vision and visual perception can represent one of the most formidably challenging aspects of neurologic consultation. This is especially true given the intimate and yet highly distributed expanse of both the anterior and posterior, as well as afferent and efferent, visual processing networks, all of which require careful and systematic examination. Even the most expert clinical assessment of the visual pathway can be augmented by technological tools such as OCT. When considering all of the highly salient limitations associated with the bedside clinical neurologic and neuro-ophthalmologic examinations, we can begin to appreciate the impact on medical practice and clinical investigation that OCT has provided.
Rapid advances in OCT imaging technology have provided unprecedented, noninvasive, in vivo imaging of the retina. Spectral-domain OCT has spectacular image resolution and very low test-retest variability. Furthermore, ultrafast acquisition rates have minimized motion artifacts, facilitated intra-subject/inter-scan co-registration with high precision, and led to post-processing algorithms for automated segmentation analyses for measurement of retinal layer thicknesses.
Considerable improvements in both the technical and practical performance characteristics of OCT have led to an exponential growth in its application to a variety of neurological diseases. This has benefited clinical practice with respect to the rapid and accurate assessment of the neuroretina, while also catalyzing a literal scientific revolution in terms of viewing the eye as a noninvasive “window” into the brain.
The neuroretina is part of the brain, comprised of first-order neurons that relay visual information to the lateral geniculate nucleus in the thalamus. While these ganglion cell layer (GCL) neurons are susceptible to primary pathologies affecting the retinal nerve fiber layer (RNFL) axons, secondary pathology that arises in other areas of the brain such as the hypothalamus and superior colliculus can also be detected in the retina.
All forms of optic neuropathy have a characteristic signature of RNFL thinning and retinal GCL atrophy that can be quantified by OCT. More remarkably, several neurodegenerative diseases with well-known brain pathology, such as Alzheimer’s disease, frontotemporal dementia, and Parkinson’s disease, are now recognized to have concomitant retinal neuronal layer degeneration that can be demonstrated by OCT. Neurological diseases traditionally linked with the peripheral nervous system are increasingly reported to have central nervous system manifestations; these include type 2a Charcot-Marie-Tooth disease, in which abnormalities in mitofusin can manifest as optic nerve disease. Deeper retinal neuronal layers, including the inner and outer nuclear layers, have been shown to be abnormal in several diseases classically associated only with primary axonal demyelination, such as MS. This supports theories that neurons may be a direct target of neurological disease processes not previously thought to have a primary degenerative mechanism. As the potential applications of OCT continue to grow, its role in neurological disease is of increasing interest and importance in discovery biology as well as in the context of clinical assessment.
In this new book we provide an extensive review of the application of OCT across a broad landscape of neurological diseases. A basic-level introduction to the underlying principles of the technology is provided for the reader. This is followed by a review of the neuroanatomy of the visual system, especially the retina, the target tissue for OCT imaging. No imaging device should be employed without proper examination of the critical link with clinical function and outcomes, most notably vision. Several chapters are dedicated to the clinical validation of the utility of OCT in neurological diseases. As expected, there are significant cross-sectional associations between retinal pathology detected by OCT and low-contrast letter acuity, high-contrast visual acuity, and color perception. Perhaps more importantly, retinal imaging appears to have predictive value for estimating cerebral pathology in gray matter structures of the central nervous system. Involvement of these structures is notoriously inconspicuous early on in many neurological diseases, and therefore an understanding of gray matter pathology may facilitate early detection and prognostication for those patients with neurodegenerative disorders. The underlying tissue pathological substrates captured with OCT-generated images are limited, and, therefore, several chapters are dedicated to elucidating the promising correlations between OCT images and histopathology, vascular pathology, as well as functional electrophysiology.
Because this new technology increasingly takes its place in the clinic, OCT has also found application in clinical trials of putative neuroprotective drugs. Indeed, the rapid thinning of the RNFL and the GCL following acute optic neuritis is a logical outcome measure for phase II trials of drugs that could exert neuroprotective or neuro-repair properties. The reproducible and accurate quantitation of the axonal and neuronal layers that undergo thinning in the three to six months following an acute optic neuritis make OCT an appealing tool, which is easily employed across multiple centers in clinical trials. Therefore, we have dedicated several chapters to reviewing the rationale, approach, and practicality of using OCT in clinical research trials. Whether retinal neuronal imaging will find the same application in neurodegenerative diseases, which manifest with changes that take place over years to decades, remains to be fully determined, but evidence supporting such a role in this context is rapidly mounting. Finally, the book concludes with a look to the future and a discussion of how the rapid evolution of this technology and associated retinal imaging devices may facilitate even more progress in structural and functional assessments of the retinal manifestations of neurological diseases. We now believe that OCT should become a mainstay of the neurological evaluation, because it is, quite simply, a quantitative ophthalmoscope.
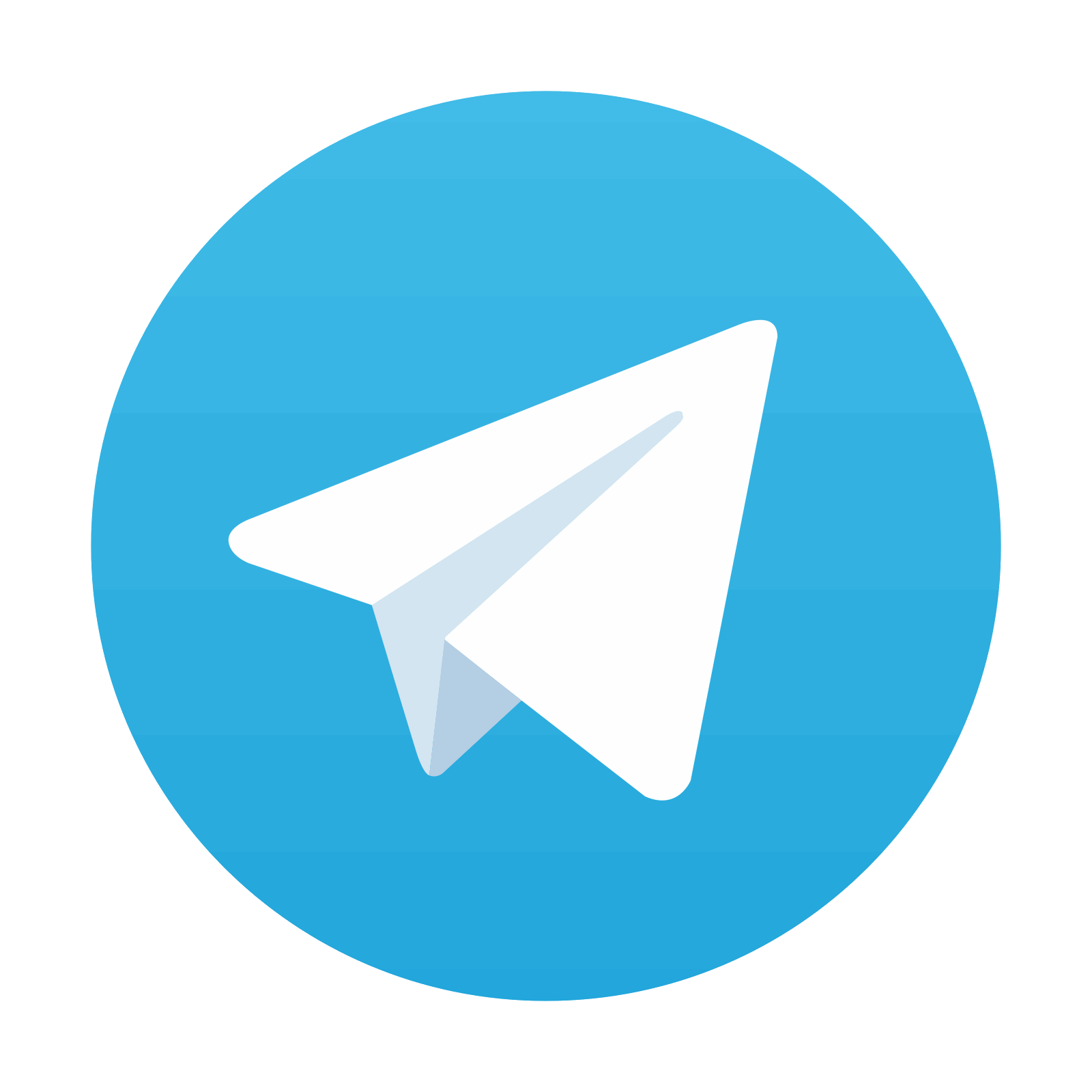
Stay updated, free articles. Join our Telegram channel

Full access? Get Clinical Tree
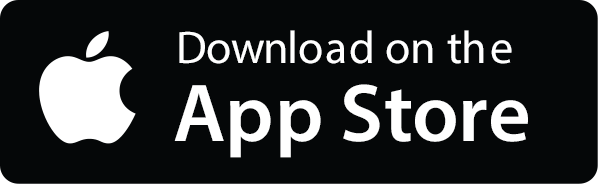
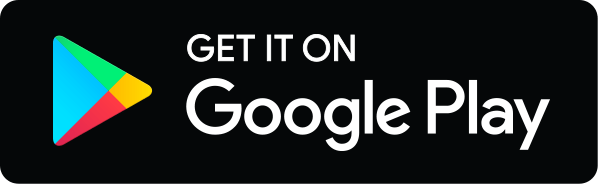