18
Management of Posterior Cranial Fossa Pathology: Gamma Knife Radiosurgery
Radiosurgery is a minimally invasive technique designed to deliver a high dose of ionizing radiation in a single treatment session using multiple beams precisely focused at the intracranial target, eliciting a specific radiobiologic response. The long-term results of radiosurgery have proven it to be an effective, minimally invasive tool in the armamentarium for the treatment of complex neurosurgical conditions. In radiosurgery, the target is not immediately destroyed or extirpated from the brain, but is instead exposed to a high dose of radiation, which ultimately results in a specific and toxic radiobiologic response, leading to arrest of the cell division, irrespective of the individual cell’s mitotic activity, oxygenation, and radiosensitivity. Radiosurgery can be performed using the gamma knife, a linear accelerator-based system, or a heavy-charged particle beam.
♦ Gamma Knife
The pioneering work on the development of the gamma knife was done at the Karolinska in Stockholm, Sweden. The most recent model is the Leksell Gamma Knife Perfexion (Elekta Instruments AB, Stockholm, Sweden) (Fig. 18.1). This model differs from previous models B and C of the Leksell gamma knife (Fig. 18.2). The 4- and 8-mm collimators remain, but the 14- and 18-mm collimators have been replaced with a 16-mm collimator.1 There is no collimator helmet, and the couch now serves as the patient positioning system.1 Doses to critical structures can be limited by a process called dynamic shaping.1 Extracranial doses are significantly lower than the previous units.1 It is now possible to treat pathology up to 26 cm caudal to the vertex of the cranium; as a result, head and neck cancers are now within the scope of gamma knife radiosurgery (GKRS).1 The previous versions of the gamma knife (models C and 4C) uses an automated positioning system (APS), eliminating the need for manual setting of the coordinates for each isocenter. Facility for image fusion is present in Leksell gamma knife model 4C.
♦ Gamma Knife Radiosurgical Procedure (Models B and C)
The gamma knife radiosurgical procedure involves four steps:
1. Application of the Leksell frame to the patient’s head
2. Stereotactic brain imaging
3. Dose planning
4. Stereotactic radiation delivery
Application of Leksell Frame
The procedure begins with rigid fixation of a magnetic resonance imaging (MRI)-compatible Leksell stereotactic frame (model G; Elekta Instruments, Atlanta, GA) to the patient’s head after careful review of the preoperative images. Frame placement is one of the most important aspects of the procedure (Fig. 18.3). The frame should be placed in such a way that the lesion should be as close to the center of the frame as possible. The scalp at the pin sites is infiltrated with local anesthesia, and the frame is secured rigidly to the outer table of the skull with pins. For lateral lesions, the frame is shifted laterally (right or left) toward the lesion. It is important to make sure that there is enough space on the contralateral side to allow positioning of the fiducial box on the frame before sending the patient for stereotactic imaging.
Stereotactic brain imaging
Magnetic resonance imaging is the preferred modality of imaging available at present for radiosurgery planning. High-resolution MRI is used for stereotactic radiosurgical planning in almost all eligible cases, except arteriovenous malformations (AVMs). The accuracy of images is checked for each image sequence by comparing the known frame measurement with image measurements. The accuracy of target localization is ensured with a properly shimmed magnet, regular servicing, and strict quality assurance on the MRI unit. A computed tomography (CT) scan is occasionally used for radiosurgical planning when MRI is contraindicated for any reason. For AVM planning, both digital subtraction angiography (DSA) and MRI images are acquired. CT cisternography can be used for treatment planning for trigeminal neuralgia if an MRI is contraindicated.
Fig. 18.1 The most recent model of the Leksell Gamma Knife Perfexion (Elekta Instruments AB, Stockholm, Sweden). Note that there is no collimator helmet, and the couch serves as patient positioning system.
Dose Planning
Stereotactic images are transported via a fiber optic Ethernet to the Gamma Plan (Elekta Instruments, Atlanta, GA) computer, where images are first checked for any distortion or inaccuracy. Planning is performed on axial MRI scans with coronal and sagittal reconstructions. Depending on the size and shape of the target volume, the appropriate collimator is used for each shot placement. The position and weightage of the shots can be adjusted to match the target volume. Every shot or isocenter has three coordinates (x, y, and z). Proper dose selection is very important for achieving conformity and for yielding a good response without any adverse effects. Shields can be used to block the radiation dose to critical structures. Various plugging patterns can be used to modify the shape. After completion of the dose-planning portion of the GKRS, it is important to perform a collision check on all shots to determine shot positions that may not be put into effect (unachievable due to the possibility of a collision between the collimator helmet, the patient’s skull, and/or any part of the frame). A neurosurgeon, radiation oncologist, and medical physicist jointly decide on the maximum dose and the dose to the margin.
Fig. 18.2 The Leksell gamma knife model C, an older model, uses an automated positioning system (APS), eliminating the need for manual setting of the coordinates for each isocenter. Unlike the Gamma Knife Perfexion model, there is a separate collimator helmet.
Fig. 18.3 The Leksell model G frame. Frame placement is one of the most important aspects of the procedure. The frame should be placed in such a way that the lesion is as close as possible to the center of the frame. For lateral lesions, the frame is shifted laterally (right or left) toward the lesion.
Stereotactic Radiation Delivery
Once the radiosurgical plan is finalized, it is exported to the control console computer and checked by the physicist. The patient is then placed comfortably on the couch in the GK-C unit, the frame is attached to the APS, and the physicist and one physician of the team perform a position and collision test run. The APS-based treatment allows the administration of a series of multiple shots (called a run) in a sequential automated session. Not all shots (shots with extreme coordinates) can be treated with APS. One may have to use the manual mode by using trunnions to treat the shots or isocenter.
Postoperative Care
All patients receive an intravenous 40-mg dose of methylprednisolone at the conclusion of the procedure. The stereotactic frame is removed after the procedure. Patients are observed for a few hours in the same-day surgical unit and are usually discharged within 24 hours.
♦ Indications for Radiosurgery in Posterior Cranial Fossa Lesions
Vestibular Schwannoma
Vestibular schwannoma are benign, slow-growing tumors that constitute approximately 10% of all primary intracranial tumors. A majority of the vestibular schwannoma grow at a rate <2 mm per year.2 Microsurgery and stereotactic radiosurgery (SRS) are well-established management options that are available to treat vestibular schwannomas. Conservative management is also an option when the tumor size is very small, when the patient states a preference for it, in patients of advanced age, and in cases in which the tumor is in the only hearing ear. The best management option for patients with small to moderate-sized vestibular schwannoma is controversial.3 However, the long-term results of SRS have established it as an important minimally invasive alternative to microsurgery.3–8 Along with long-term tumor control, other goals of GKRS treatment are hearing preservation and preservation of facial nerve function and trigeminal nerve function. Facial nerve preservation rates of approximately 95 to 100% have been reported with SRS.3,6,8,9 Hearing preservation rates of 50 to 75% have been reported with SRS.6,10,11
Radiosurgical Technique for Acoustic Tumors
♦ Preradiosurgical Evaluations
Radiologic evaluation should include high-resolution thin-section MRI scans. Hearing is graded using the Gardner-Robertson modification of the Silverstein and Norell classification,12 and facial nerve function is assessed according to the House-Brackmann grading system.13 Serviceable hearing (classes I and II) is defined as a pure tone average (PTA) or speech reception threshold lower than 50 dB and an speech discrimination score (SDS) better than 50%. Patients need to be counseled about various treatment options including conservative treatment. Occasionally in patients with neurofibromatosis, one may need to do a trial fitting of the frame.
♦ Radiosurgical Technique: Frame Placement
The aim of the frame placement is to bring the lesion as close to the center as possible. This can be achieved with placing the frame as low as possible and shifting it backward. The anterior posts are positioned as low as possible on the supra-orbital region to avoid collision of the frontal post or pin with the collimator helmet. Sometimes in muscular individuals, it might be difficult to secure the frame with short posterior posts; in such cases long posts can be used to secure the frame posteriorly. This might minimize the pain, as the pins will travel through less suboccipital muscles.
♦ Dose Planning
The dose plan should be highly conformal especially at the anterior margin of the tumor, where the facial nerve and the cochlear nerve complex generally are located.
♦ Dose Prescription
The accepted marginal dose is 13 Gy. With this dose lower complication rates with good tumor control have been re-ported.14 Lower marginal doses are prescribed for patients with bilateral acoustic neuroma (neurofibromatosis type 2 [NF2]) or for patients with contralateral deafness, for whom hearing preservation may be more critical.
♦ Outcome of Gamma Knife Radiosurgery of Acoustic Schwannoma
In the study by Pollock et al,3 early outcomes were better for patients having SRS compared with surgical resection (level 2 evidence). The need for later tumor resection following SRS is less than 3%.3 In the study by Banerjee et al,15 radiosurgery is less expensive than microsurgical resection. Facial nerve preservation rates of approximately 95 to 100% have been reported with SRS.3,6,8,9 The treatment dose appears to be much more important than the treatment plan quality in the prevention of facial numbness or weakness after radiosurgery for vestibular schwannoma.16 Hearing preservation rates of 50 to 75% have been reported with SRS.6,10,11 A high dose of radiation to the inner ear structures could be a cause for hearing loss after radiosurgery.17 In the study by Massager et al,18 the mean dose of radiation to the cochlea was 4.33 Gy (range 1.30 to 10.00 Gy), and they found that there is a significant correlation between the radiation dose received by the cochlea and the audiologic outcome. In another study, a maximum radiotherapy dose to the cochlear nucleus of >10 Gy was associated with hearing deterioration.19 Unlike microsurgery, hearing loss is gradual over 6 to 24 months. The hearing deterioration after radiosurgery could be due to radiation damage to the cochlear apparatus, disruption in the blood supply to the cochlea, adhesion between the tumor and the perineural tissue, a volume change of the tumor located in the internal auditory canal (IAC), and the compression effect on the cochlear nerve.20–22
♦ Surgery After Gamma Knife Radiosurgery of Acoustic Schwannoma
The need for later tumor resection following SRS is less than 3%.3 There are reports in which tumor removal after failed SRS is difficult due to fibrosis and adhesions.20,23 Others did not find any adhesion between the tumor and facial nerve after SRS.24 Transient swelling following SRS is observed in 62% of cases treated with SRS, and surgical excision needs to be carefully considered because of the natural regression of transient tumor swelling over time.25 Because of the high rates of facial nerve dysfunction following surgery for failed SRS treatment,20,26 subtotal resection of the tumor may facilitate preservation of cranial nerve function.23,25
♦ Acoustic Schwannoma and Neurofibromatosis Type 2
The local control following SRS of vestibular schwannoma in the context of NF2 varies between 74% and 100%.27–30 In two of the larger series there were no concerns regarding radiosurgery-associated secondary tumors in patients with vestibular schwannoma in the context of NF228,30; however, all patients need to be informed about such events.
Trigeminal Schwannoma
Trigeminal nerve schwannomas account for 0.2 to 1% of all intracranial tumors and 0.8 to 8% of intracranial schwannomas. Schwannomas are rare and slow growing, and surgery is the primary treatment option. In a recent review the rate of trigeminal nerve dysfunction (new/worsened) after lesion removal varies from 13–86%.31 Radiosurgery may be a preferable alternative to surgical removal, especially for small to medium-sized tumors, because of its low morbidity. Tumor recurrence following microsurgery is similar to the tumor progression rate after radiosurgery.32–34 The accepted marginal dose is between 13 and 14 Gy, which is adequate to control trigeminal schwannoma locally. The overall tumor control reported in various studies is 90 to 100%,33–36 with a relatively less incidence of new or worsening of symptoms compared with microsurgical approach. The incidence of new-onset cranial neuropathies range between 0 and 30%,33,34,36 and the lack of central enhancement could be a warning sign of cranial neuropathies.36
Meningioma
Posterior cranial fossa meningiomas constitute approximately 9% of all intracranial meningiomas. The most common surgical sequela is new or worsening cranial nerve deficit. The incidence of facial nerve weakness following posterior fossa meningioma surgery varies from 10.5 to 30%.37 The incidence of cerebrospinal fluid (CSF) leak following posterior fossa meningioma excision varies from 2.5 to 13.6%, and gross total resection varies from 57 to 94%.38–40 Within the past few years, radiosurgery has played an increasingly important role as a minimally invasive therapeutic modality, not only as an adjunctive but also as a primary treatment. The optimal dose for meningiomas is still under debate.41 In recently published studies the marginal dose varied between 12 and 20 Gy.41–45 In some of the above studies the 5-year progression-free survival after GKRS for meningiomas varied from 93 to 98%,41,46,47 with a marginal dose of 12 to 13 Gy. In one of the largest study of GKRS for meningiomas by Kollova et al,41 a marginal dose greater than 16 Gy was associated with edema, and with marginal dose of less than 12 Gy there was a higher incidence of an increase in tumor volume after radiosurgery. In older patients, in patients with significant concomitant medical problems or a high-risk tumor location, and in patients who are not willing to undergo an open microsurgical procedure, we would recommend performing radiosurgery as a safe and effective alternative primary treatment modality. Figure 18.4 shows the radiosurgical planning for a petroclival meningioma. The plan needs to be highly conformal toward the brainstem.
Chordoma and Chondrosarcoma
Seven percent of chondrosarcomas48 originate from the posterior fossa and 35% of chordomas originate from the spheno-occipital area. Surgical excision is the primary modality to treat these lesions. Because of the close proximity to critical neurovascular structures, gross total resection is rarely achieved. In addition, most patients require postoperative radiotherapy. Tzortzidis et al49 reported a 42% recurrence-free survival for primary cases (microsurgery) and 26% recurrence-free survival for reoperated cases at 10-year follow-up for chordoma. In one of the studies with the longest follow-up period for cranial chondrosarcomas,50 the authors reported a recurrence-free survival of 32% at 10-year follow-up following microsurgical resection. In one of the largest radiosurgical series,51 the 5-and 10-year local tumor control rates were 87% and 76%, respectively, in patients with small to medium-sized tumors of less than 20 mL. These authors recommend a marginal dose of 15 Gy to achieve long-term control. When a tumor residual volume is 20 mL or greater, even after maximum debulking efforts, charged-particle radiotherapy is recommended.51 Charged-particle radiotherapy (proton-beam radiotherapy) can achieve a substantial reduction in the volume of irradiated nontarget brain tissues. The beneficial impact of this radio therapeutic modality can be attributed to the known Bragg peak effect: protons lose energy at an increasing rate as they slow down in traversed tissue, yielding an enhanced region of energy deposition—the Bragg peak—just before they stop.
Fig. 18.4 Gamma knife treatment plan for a case of petroclival meningioma, the plan shows axial and reconstructed MRI images. The lines within the treatment plan represent isodose lines and shows the steep radiation dose fall-off.
Glomus Tumor
One of the practical problems in treating the glomus jugulare tumors is that the frame should be fixed as low as possible. The mainstay of treatment is surgical resection or external beam radiotherapy or both. The results of GKRS have encouraged centers to use SRS in the treatment of glomus jugulare tumors. GKRS can be used to treat these lesions primarily or can be used to treat any residual or recurrent lesions. In one study with a median follow-up of 7.2 years, tumor control rate was 94.7%.52 Pollock et al53 reported a progression-free survival of 75% at 10 years. Even the short-term results are very encouraging. In recently published reports, tumor control rates of 100% have been reported at a median follow-up of greater than 24 months.54,55 Proper selection of the patients is important. A marginal dose of 16 Gy (range 12–18 Gy) is generally well tolerated and effective.53,54 Proximity to brainstem and cranial nerves might dictate a reduction in the marginal dose.
Hemangioblastoma
Microsurgical resection offers cure in the majority of cases. Vascularity of the tumors and location in critical areas of the brain are the common reasons why in some patients completion resection is impossible. In multiple lesions, the advantage of the gamma knife is that the lesions can all be treated simultaneously. GKRS may not be an ideal choice for cystic hemangioblastoma, especially with large cysts.56–58 The most accepted marginal dose is approximately 18 Gy.
Malignant Glioma
All the studies so far had evaluated the role of GKRS as an adjuvant treatment rather than primary modality. Souhami et al,59 in their multicentre randomized trial, did not find any beneficial effect in adding SRS to the initial management of glioblastoma multiforme (GBM). Though the results of some studies are encouraging,60,61 caution needs to exercised as the patients treated in the above series were a highly selective group. In the published literature, there was no uniformity in dose selection.62
Low-Grade Glioma
Experiences in treating low-grade glioma with radiosurgery are limited. Several small studies have demonstrated early efficacy of radiosurgery in the setting of low-grade glioma.63–65 However, prolonged follow-up assessing long-term control in a large series is required.
Brainstem Glioma
For diffuse brainstem glioma, radiosurgery is not appropriate.66 Typically, such largely dispersed lesions manifest an aggressive biologic course and indicate a most serious prognosis. Neither surgery nor radiotherapy including GKRS is considered fitting. Radiosurgery is one of the options for small focal lesions of the brainstem as a primary treatment or following surgical decompression,66,67 but these studies have limitations such as small numbers of patients, no uniform consensus regarding the dose, and short follow-up period. The usual accepted brainstem tolerance dose is 12 Gy. 68
Medulloblastoma
The evidence for using GKRS in the management of unresectable residual or recurrent tumor comes from very few studies.69,70 With the growing experience with radiosurgery, more long-term outcome data may become available in the future to support its role in the management of these tumors.
Metastasis
The regional distribution of the metastasis in the brain is reported to be 80 to 85% in the cerebral hemispheres, 10 to 15% in the cerebellum, and 3 to 5% in the brainstem.71 According to the review by Mintz et al,72 two randomized trials73,74 demonstrated a significant survival benefit for patients who received surgery plus whole-brain radiation therapy (WBRT) as compared with patients who received WBRT alone, and one randomized trial detected no significant survival difference between the treatment groups.75
Surgery followed by WBRT is recommended for patients with good performance status, minimal or no evidence of extracranial disease, and a surgically accessible single brain metastasis amenable to complete excision.72 The optimal dose and fractionation schedule for WBRT is 3000 cGy in 10 fractions. In a recently published randomized controlled trial (RCT)76 there was no difference in survival rates between GKRS alone and surgery plus WBRT for a single small brain metastasis.
For a single surgically unresectable metastasis, the results of a radiosurgery boost after WBRT are better than WBRT alone.77 For one to four brain metastases, in one RCT there was no improvement in survival, with WBRT plus SRS when compared with SRS alone.78 According to the Radiation Therapy Oncology Group (RTOG) trial 9508,77 the radiosurgery boost after WBRT for a single unresectable metastasis was better than WBRT alone, and this strategy is to be considered for two or three brain metastases as well.
Two factors that influence the marginal dose are the tumor size and previous radiotherapy.79 In the literature the prescribed marginal dose varied between 13 and 20 Gy.79–83 The most frequently prescribed marginal dose is 16 Gy.
Arteriovenous Malformations
Posterior cranial fossa AVMs, which receive arterial feeders from the vertebrobasilar system, constitute approximately 10% of all AVMs. Patients presenting with hemorrhage from posterior fossa AVMs have a significant risk of rehemorrhage (8.4–9.4% per year).84 GKRS is a well-established technique to cure AVMs of the brain. The disadvantages with GKRS are that the results are not instantaneous, unlike with surgical excision. There is always a risk of rebleeding during the waiting period. The risk of rebleeding after radiosurgery varied from 3.4 to 10%.85 Radiosurgery is appropriate for patients with small AVMs, especially when they are located in eloquent brain locations. The volume of the nidus is a more important factor than the maximum diameter.86 Treatment planning requires acquisition of thin-section MRI scans and digital subtraction angiograms. In cases where MRI is contraindicated, a CT angiogram can be performed. Conformity should be based on both MRI scans and DSA. The optimal marginal dose frequently prescribed is 25 Gy. A dose reduction may be necessary when the AVM is large or the nidus is not compact and located in the eloquent region of the brain. Radiosurgery can eliminate the risk of hemorrhage in approximately 75% of all AVM patients within 3 years of the procedure and can be repeated after 3 years.87,88 Individual obliteration rates vary from 50 to 88% depending on the marginal dose.89 Even for larger AVMs, staged volume radiosurgery is proposed.90,91 Radiosurgical planning for a small AVM in the vermian region is shown in Fig. 18.5.
Fig. 18.5 Radiosurgical planning for a small AVM located in the vermian region.
Brainstem Arteriovenous Malformations
Although surgery is advisable for brainstem AVMs reaching the pial surface, there is always a risk of causing neurologic damage in performing such an excision. Deeply located brainstem AVMs are best treated with radiosurgery. The compact nature of brainstem parenchyma, having critical neurologic function, undoubtedly increases both the radiosurgical and microsurgical complication rates. Conformal dose planning and appropriate dose selections are keys to the reduction of adverse radiation effects. Because the optimal dose is determined on the balance between the expected obliteration rates and the corresponding risks of radiation injury, knowledge of tolerable doses is essential in planning radiosurgical treatment.
♦ Cavernous Malformations
Asymptomatic cavernous malformations can be treated conservatively. Intervention is required in cases with intractable seizures, recurrent hemorrhages, and hemorrhage causing severe neurologic deficits.92 For lesions that are located superficially and in noneloquent regions, surgical excision is safe and the risk of rebleeding can be eliminated. The role of radiosurgery in the management of cavernomas is controversial. Radiosurgery of the cavernomas is indicated for poorly accessible lesions such as brainstem cavernomas, where microsurgery is considered risky.92
Currently, the marginal dose for cavernous hemangioma in our practice does not exceed 15 Gy, even for small lesions. The theoretical goal of radiosurgery is to obliterate the lesion completely and thereby to prevent subsequent rebleeding. Unlike cerebral AVMs, direct proof of the postradiosurgical obliteration of these lesions is not available. There are difficulties in defining what constitutes a cavernous hemangioma bleeding93 and evaluating the results of GKRS. GKRS does not remove the intracranial pathologic process completely. The lesion regression and a decrease in volume can be regarded as a positive treatment response. The only way to evaluate the risk of rebleeding is clinical observation during a longer follow-up period. Thus, a longer follow-up period is needed to verify the protective effect of the radiosurgery on cavernous hemangioma. Lesion regression or failure to enlarge is an important parameter in relation to the effect of GKRS on a cavernous hemangioma.
Trigeminal Neuralgia
More recently, radiosurgery has emerged as a viable treatment option for trigeminal neuralgia.94–96 Patients are often offered medical therapy as the initial line of treatment. However, many patients fail or cannot tolerate medical therapy, and eventually require surgical intervention. Although often associated with initial pain relief, all surgical procedures have variable but definite rates of recurrence and morbidity. The addition of radiosurgery to the treatment armamentarium offers patients another less invasive alternative. Radiosurgery has been advocated as a minimally invasive alternative surgical approach to microvascular decompression (MVD) or percutaneous procedures. A single 4-mm isocenter is used for targeting the trigeminal root entry zone. The target is usually 3- to 6-mm anterior to the pontine edge (Fig. 18.6). The most accepted maximal dose is 80 Gy. Excellent outcomes were achieved within the first several weeks after the procedure in 35 to 74% of patients.97–99 The latency interval to pain relief is approximately 1 to 2 months. One of the most significant advantages of radiosurgery is the low risk of new trigeminal dysfunction, compared with other percutaneous surgical techniques. The incidence of this complication after radiosurgery has been reported to be between 0 and 17%.97–99 Radiosurgery may be appropriate for a subset of patients who are not good surgical candidates. In addition, radiosurgery could be considered a good choice for patients with recurrent pain after failure of MVD or percutaneous surgery. Radiosurgery can be repeated if pain returns after initial relief. At the second procedure the maximum dose is reduced (60–70 Gy) and the nerve is targeted anterior to the prior target.100
Fig. 18.6 Radiosurgical planning for a trigeminal neuralgia. A single 4-mm isocenter is used for targeting the trigeminal root entry zone. The target is usually 3 to 6 mm anterior to the pontine edge.
References
1. Lindquist C, Paddick I. The Leksell Gamma Knife Perfexion and comparisons with its predecessors. Neurosurgery 2007;61(3, Suppl):130– 140, discussion 140–141 PubMed
2. Raut VV, Walsh RM, Bath AP, et al. Conservative management of vestibular schwannomas—second review of a prospective longitudinal study. Clin Otolaryngol Allied Sci 2004;29:505–514 PubMed
3. Pollock BE, Driscoll CL, Foote RL, et al. Patient outcomes after vestibular schwannoma management: a prospective comparison of microsurgical resection and stereotactic radiosurgery. Neurosurgery 2006;59:77–85, discussion 77–85 PubMed
4. Flickinger JC, Kondziolka D, Niranjan A, Lunsford LD. Results of acoustic neuroma radiosurgery: an analysis of 5 years’ experience using current methods. J Neurosurg 2001;94:1–6 PubMed
5. Régis J, Pellet W, Delsanti C, et al. Functional outcome after gamma knife surgery or microsurgery for vestibular schwannomas. J Neurosurg 2002;97:1091–1100 PubMed
6. Chopra R, Kondziolka D, Niranjan A, Lunsford LD, Flickinger JC. Long-term follow-up of acoustic schwannoma radiosurgery with marginal tumor doses of 12 to 13 Gy. Int J Radiat Oncol Biol Phys 2007;68:845–851 PubMed
7. Hasegawa T, Kida Y, Kobayashi T, Yoshimoto M, Mori Y, Yoshida J. Long-term outcomes in patients with vestibular schwannomas treated using gamma knife surgery: 10-year follow up. J Neurosurg 2005;102:10–16 PubMed
8. Lunsford LD, Niranjan A, Flickinger JC, Maitz A, Kondziolka D. Radiosurgery of vestibular schwannomas: summary of experience in 829 cases. J Neurosurg 2005;102(Suppl):195–199 PubMed
9. Chung WY, Liu KD, Shiau CY, et al. Gamma knife surgery for vestibular schwannoma: 10-year experience of 195 cases. J Neurosurg 2005;102 (Suppl):87–96 PubMed
10. Chang SD, Gibbs IC, Sakamoto GT, Lee E, Oyelese A, Adler JR Jr. Staged stereotactic irradiation for acoustic neuroma. Neurosurgery 2005;56: 1254–1261, discussion 1261–1263 PubMed
11. Hasegawa T, Fujitani S, Katsumata S, Kida Y, Yoshimoto M, Koike J. Stereotactic radiosurgery for vestibular schwannomas: analysis of 317 patients followed more than 5 years. Neurosurgery 2005;57:257–265, discussion 257–265 PubMed
12. Gardner G, Robertson JH. Hearing preservation in unilateral acoustic neuroma surgery. Ann Otol Rhinol Laryngol 1988;97:55–66 PubMed
13. House JW, Brackmann DE. Facial nerve grading system. Otolaryngol Head Neck Surg 1985;93:146–147 PubMed
14. Flickinger JC, Kondziolka D, Pollock BE, Lunsford LD. Evolution in technique for vestibular schwannoma radiosurgery and effect on outcome. Int J Radiat Oncol Biol Phys 1996;36:275–280 PubMed
15. Banerjee R, Moriarty JP, Foote RL, Pollock BE. Comparison of the surgical and follow-up costs associated with microsurgical resection and stereotactic radiosurgery for vestibular schwannoma. J Neurosurg 2008; 108:1220–1224 PubMed
16. Beegle RD, Friedman WA, Bova FJ. Effect of treatment plan quality on outcomes after radiosurgery for vestibular schwannoma. J Neurosurg 2007;107:913–916 PubMed
17. Linskey ME, Johnstone PA, O’Leary M, Goetsch S. Radiation exposure of normal temporal bone structures during stereotactically guided gamma knife surgery for vestibular schwannomas. J Neurosurg 2003;98:800– 806 PubMed
18. Massager N, Nissim O, Delbrouck C, et al. Irradiation of cochlear structures during vestibular schwannoma radiosurgery and associated hearing outcome. J Neurosurg 2007;107:733–739 PubMed
19. Paek SH, Chung HT, Jeong SS, et al. Hearing preservation after gamma knife stereotactic radiosurgery of vestibular schwannoma. Cancer 2005;104:580–590 PubMed
20. Lee DJ, Westra WH, Staecker H, Long D, Niparko JK, Slattery WH III. Clinical and histopathologic features of recurrent vestibular schwannoma (acoustic neuroma) after stereotactic radiosurgery. Otol Neurotol 2003;24:650–660, discussion 660 PubMed
21. Nakamura H, Jokura H, Takahashi K, Boku N, Akabane A, Yoshimoto T. Serial follow-up MR imaging after gamma knife radiosurgery for vestibular schwannoma. AJNR Am J Neuroradiol 2000;21:1540–1546 PubMed
22. Yu CP, Cheung JY, Leung S, Ho R. Sequential volume mapping for confirmation of negative growth in vestibular schwannomas treated by gamma knife radiosurgery. J Neurosurg 2000;93(Suppl 3):82–89 PubMed
23. Shuto T, Inomori S, Matsunaga S, Fujino H. Microsurgery for vestibular schwannoma after gamma knife radiosurgery. Acta Neurochir (Wien) 2008;150:229–234, discussion 234 PubMed
24. Kwon Y, Khang SK, Kim CJ, Lee DJ, Lee JK, Kwun BD. Radiologic and histopathologic changes after Gamma Knife radiosurgery for acoustic schwannoma. Stereotact Funct Neurosurg 1999;72(Suppl 1):2–10 PubMed
25. Iwai Y, Yamanaka K, Yamagata K, Yasui T. Surgery after radiosurgery for acoustic neuromas: surgical strategy and histological findings. Neurosurgery 2007;60(2, Suppl 1):ONS75–ONS82, discussion ONS82 PubMed
26. Schulder M, Sreepada GS, Kwartler JA, Cho ES. Microsurgical removal of a vestibular schwannoma after stereotactic radiosurgery: surgical and pathologic findings. Am J Otol 1999;20:364–367, discussion 368 PubMed
27. Kida Y, Kobayashi T, Tanaka T, Mori Y. Radiosurgery for bilateral neurinomas associated with neurofibromatosis type 2. Surg Neurol 2000; 53:383–389, discussion 389–390 PubMed
28. Mathieu D, Kondziolka D, Flickinger JC, et al. Stereotactic radiosurgery for vestibular schwannomas in patients with neurofibromatosis type 2: an analysis of tumor control, complications, and hearing preservation rates. Neurosurgery 2007;60:460–468, discussion 468–470 PubMed
29. Roche PH, Régis J, Pellet W, et al. Neurofibromatosis type 2. Preliminary results of gamma knife radiosurgery of vestibular schwannomas. Neurochirurgie 2000;46:339–353, discussion 354 PubMed
30. Rowe JG, Radatz MW, Walton L, Soanes T, Rodgers J, Kemeny AA. Clinical experience with gamma knife stereotactic radiosurgery in the management of vestibular schwannomas secondary to type 2 neurofibromatosis. J Neurol Neurosurg Psychiatry 2003;74:1288–1293 PubMed
31. Kano H, Niranjan A, Kondziolka D, Flickinger JC, Dade Lunsford L. Stereotactic radiosurgery for trigeminal schwannoma: tumor control and functional preservation. Clinical article. J Neurosurg 2009;110(3):553–558.
32. Goel A, Muzumdar D, Raman C. Trigeminal neuroma: analysis of surgical experience with 73 cases. Neurosurgery 2003;52:783–790, discussion 790 PubMed
33. Nettel B, Niranjan A, Martin JJ, et al. Gamma knife radiosurgery for trigeminal schwannomas. Surg Neurol 2004;62:435–444, discussion 444– 446 PubMed
34. Pollock BE, Foote RL, Stafford SL. Stereotactic radiosurgery: the preferred management for patients with nonvestibular schwannomas? Int J Radiat Oncol Biol Phys 2002;52:1002–1007 PubMed
35. Pan L, Wang EM, Zhang N, et al. Long-term results of Leksell gamma knife surgery for trigeminal schwannomas. J Neurosurg 2005;102 (Suppl):220–224 PubMed
36. Phi JH, Paek SH, Chung HT, et al. Gamma Knife surgery and trigeminal schwannoma: is it possible to preserve cranial nerve function? J Neurosurg 2007;107:727–732 PubMed
37. Samii M, Tatagiba M. Experience with 36 surgical cases of petroclival meningiomas. Acta Neurochir (Wien) 1992;118:27–32 PubMed
38. Lobato RD, Gonzaáez P, Alday R, et al. Meningiomas of the basal posterior fossa. Surgical experience in 80 cases. Neurocirugia (Astur) 2004; 15:525–542 PubMed
39. Roberti F, Sekhar LN, Kalavakonda C, Wright DC. Posterior fossa meningiomas: surgical experience in 161 cases. Surg Neurol 2001;56:8–20, discussion 20–21 PubMed
40. Shen T, Friedman RA, Brackmann DE, et al. The evolution of surgical approaches for posterior fossa meningiomas. Otol Neurotol 2004;25: 394–397 PubMed
41. Kollová A, Liscák R, Novotný J Jr, Vladyka V, Simonová G, Janousková L. Gamma Knife surgery for benign meningioma. J Neurosurg 2007;107: 325–336 PubMed
42. DiBiase SJ, Kwok Y, Yovino S, et al. Factors predicting local tumor control after gamma knife stereotactic radiosurgery for benign intracranial meningiomas. Int J Radiat Oncol Biol Phys 2004;60:1515–1519 PubMed
43. Kim DG, Kim ChH, Chung HT, et al. Gamma knife surgery of superficially located meningioma. J Neurosurg 2005;102(Suppl):255–258 PubMed
44. Linskey ME, Davis SA, Ratanatharathorn V. Relative roles of microsurgery and stereotactic radiosurgery for the treatment of patients with cranial meningiomas: a single-surgeon 4-year integrated experience with both modalities. J Neurosurg 2005;102(Suppl):59–70 PubMed
45. Malik I, Rowe JG, Walton L, Radatz MW, Kemeny AA. The use of stereotactic radiosurgery in the management of meningiomas. Br J Neurosurg 2005;19:13–20 PubMed
46. Kreil W, Luggin J, Fuchs I, Weigl V, Eustacchio S, Papaefthymiou G. Long term experience of gamma knife radiosurgery for benign skull base meningiomas. J Neurol Neurosurg Psychiatry 2005;76:1425–1430 PubMed
47. Lee JY, Niranjan A, McInerney J, Kondziolka D, Flickinger JC, Lunsford LD. Stereotactic radiosurgery providing long-term tumor control of cavernous sinus meningiomas. J Neurosurg 2002;97:65–72 PubMed
48. Barnes L, Kapadia SB. The biology and pathology of selected skull base tumors. J Neurooncol 1994;20:213–240 PubMed
49. Tzortzidis F, Elahi F, Wright D, Natarajan SK, Sekhar LN. Patient outcome at long-term follow-up after aggressive microsurgical resection of cranial base chordomas. Neurosurgery 2006;59:230–237, discussion 230–237 PubMed
50. Tzortzidis F, Elahi F, Wright DC, Temkin N, Natarajan SK, Sekhar LN. Patient outcome at long-term follow-up after aggressive microsurgical resection of cranial base chondrosarcomas. Neurosurgery 2006;58: 1090–1098, discussion 1090–1098 PubMed
51. Martin JJ, Niranjan A, Kondziolka D, Flickinger JC, Lozanne KA, Lunsford LD. Radiosurgery for chordomas and chondrosarcomas of the skull base. J Neurosurg 2007;107:758–764 PubMed
52. Eustacchio S, Trummer M, Unger F, Schröttner O, Sutter B, Pendl G. The role of Gamma Knife radiosurgery in the management of glomus jugular tumours. Acta Neurochir Suppl (Wien) 2002;84:91–97 PubMed
53. Pollock BE. Stereotactic radiosurgery in patients with glomus jugulare tumors. Neurosurg Focus 2004;17:E10 PubMed
54. Sharma MS, Gupta A, Kale SS, Agrawal D, Mahapatra AK, Sharma BS. Gamma knife radiosurgery for glomus jugulare tumors: therapeutic advantages of minimalism in the skull base. Neurol India 2008;56:57– 61 PubMed
55. Sheehan J, Kondziolka D, Flickinger J, Lunsford LD. Gamma knife surgery for glomus jugulare tumors: an intermediate report on efficacy and safety. J Neurosurg 2005;102(Suppl):241–246 PubMed
56. Matsunaga S, Shuto T, Inomori S, Fujino H, Yamamoto I. Gamma knife radiosurgery for intracranial haemangioblastomas. Acta Neurochir (Wien) 2007;149:1007–1013, discussion 1013 PubMed
57. Tago M, Terahara A, Shin M, et al. Gamma knife surgery for hemangioblastomas. J Neurosurg 2005;102(Suppl):171–174 PubMed
58. Wang EM, Pan L, Wang BJ, et al. The long-term results of gamma knife radiosurgery for hemangioblastomas of the brain. J Neurosurg 2005; 102(Suppl):225–229 PubMed
59. Souhami L, Seiferheld W, Brachman D, et al. Randomized comparison of stereotactic radiosurgery followed by conventional radiotherapy with carmustine to conventional radiotherapy with carmustine for patients with glioblastoma multiforme: report of Radiation Therapy Oncology Group 93-05 protocol. Int J Radiat Oncol Biol Phys 2004;60:853–860 PubMed
60. Combs SE, Widmer V, Thilmann C, Hof H, Debus J, Schulz-Ertner D. Stereotactic radiosurgery (SRS): treatment option for recurrent glioblastoma multiforme (GBM). Cancer 2005;104:2168–2173 PubMed
61. Nwokedi EC, DiBiase SJ, Jabbour S, Herman J, Amin P, Chin LS. Gamma knife stereotactic radiosurgery for patients with glioblastoma multiforme. Neurosurgery 2002;50:41–46, discussion 46–47 PubMed
62. Crowley RW, Pouratian N, Sheehan JP. Gamma knife surgery for glioblastoma multiforme. Neurosurg Focus 2006;20:E17 PubMed
63. Boëthius J, Ulfarsson E, Rähn T, Lippittz B. Gamma knife radiosurgery for pilocytic astrocytomas. J Neurosurg 2002;97(5, Suppl):677–680 PubMed
64. Hadjipanayis CG, Niranjan A, Tyler-Kabara E, Kondziolka D, Flickinger JC, Lunsford LD. Stereotactic radiosurgery for well-circumscribed fibrillary grade II astrocytomas: an initial experience. Stereotact Funct Neurosurg 2002;79:13–24 PubMed
65. Heppner PA, Sheehan JP, Steiner LE. Gamma knife surgery for low-grade gliomas. Neurosurgery 2005;57:1132–1139 discussion PubMed
66. Yen CP, Sheehan J, Steiner M, Patterson G, Steiner L. Gamma knife surgery for focal brainstem gliomas. J Neurosurg 2007;106:8–17 PubMed
67. Fuchs I, Kreil W, Sutter B, Papaethymiou G, Pendl G. Gamma Knife radiosurgery of brainstem gliomas. Acta Neurochir Suppl (Wien) 2002; 84:85–90 PubMed
68. Perks JR, St George EJ, El Hamri K, Blackburn P, Plowman PN. Stereotactic radiosurgery XVI: isodosimetric comparison of photon stereotactic radiosurgery techniques (gamma knife vs. micromultileaf collimator linear accelerator) for acoustic neuroma—and potential clinical importance. Int J Radiat Oncol Biol Phys 2003;57:1450–1459 PubMed
69. Germanwala AV, Mai JC, Tomycz ND, et al. Boost Gamma Knife surgery during multimodality management of adult medulloblastoma. J Neurosurg 2008;108:204–209 PubMed
70. Hodgson DC, Goumnerova LC, Loeffler JS, et al. Radiosurgery in the management of pediatric brain tumors. Int J Radiat Oncol Biol Phys 2001;50:929–935 PubMed
71. Delattre JY, Krol G, Thaler HT, Posner JB. Distribution of brain metastases. Arch Neurol 1988;45:741–744 PubMed
72. Mintz A, Perry J, Spithoff K, Chambers A, Laperriere N. Management of single brain metastasis: a practice guideline. Curr Oncol 2007;14:131– 143 PubMed
73. Patchell RA, Tibbs PA, Walsh JW, et al. A randomized trial of surgery in the treatment of single metastases to the brain. N Engl J Med 1990; 322:494–500 PubMed
74. Vecht CJ, Haaxma-Reiche H, Noordijk EM, et al. Treatment of single brain metastasis: radiotherapy alone or combined with neurosurgery? Ann Neurol 1993;33:583–590 PubMed
75. Mintz AH, Kestle J, Rathbone MP, et al. A randomized trial to assess the efficacy of surgery in addition to radiotherapy in patients with a single cerebral metastasis. Cancer 1996;78:1470–1476 PubMed
76. Muacevic A, Wowra B, Siefert A, Tonn JC, Steiger HJ, Kreth FW. Microsurgery plus whole brain irradiation versus Gamma Knife surgery alone for treatment of single metastases to the brain: a randomized controlled multicentre phase III trial. J Neurooncol 2008;87:299–307 PubMed
77. Andrews DW, Scott CB, Sperduto PW, et al. Whole brain radiation therapy with or without stereotactic radiosurgery boost for patients with one to three brain metastases: phase III results of the RTOG 9508 randomised trial. Lancet 2004;363:1665–1672 PubMed
78. Aoyama H, Shirato H, Tago M, et al. Stereotactic radiosurgery plus whole-brain radiation therapy vs stereotactic radiosurgery alone for treatment of brain metastases: a randomized controlled trial. JAMA 2006; 295:2483–2491 PubMed
79. Lorenzoni JG, Devriendt D, Massager N, et al. Brain stem metastases treated with radiosurgery: prognostic factors of survival and life expectancy estimation. Surg Neurol 2009;71:188–195, discussion 195, 195– 196 PubMed
80. Fuentes S, Delsanti C, Metellus P, Peragut JC, Grisoli F, Regis J. Brainstem metastases: management using gamma knife radiosurgery. Neurosurgery 2006;58:37–42, discussion 37–42 PubMed
81. Hussain A, Brown PD, Stafford SL, Pollock BE. Stereotactic radiosurgery for brainstem metastases: Survival, tumor control, and patient outcomes. Int J Radiat Oncol Biol Phys 2007;67:521–524 PubMed
82. Kased N, Huang K, Nakamura JL, et al. Gamma knife radiosurgery for brainstem metastases: the UCSF experience. J Neurooncol 2008;86: 195–205 PubMed
83. Yen CP, Sheehan J, Patterson G, Steiner L. Gamma knife surgery for metastatic brainstem tumors. J Neurosurg 2006;105:213–219 PubMed
84. Kelly ME, Guzman R, Sinclair J, et al. Multimodality treatment of posterior fossa arteriovenous malformations. J Neurosurg 2008;108:1152– 1161 PubMed
85. Liscák R, Vladyka V, Simonová G, et al. Arteriovenous malformations after Leksell gamma knife radiosurgery: rate of obliteration and complications. Neurosurgery 2007;60:1005–1014, discussion 1015–1016 PubMed
86. Karlsson B, Lindquist C, Steiner L. Prediction of obliteration after gamma knife surgery for cerebral arteriovenous malformations. Neurosurgery 1997;40:425–430, discussion 430–431 PubMed
87. Seifert V, Stolke D, Mehdorn HM, Hoffmann B. Clinical and radiological evaluation of long-term results of stereotactic proton beam radiosurgery in patients with cerebral arteriovenous malformations. J Neurosurg 1994;81:683–689 PubMed
88. Steinberg GK, Chang SD, Gewirtz RJ, Lopez JR. Microsurgical resection of brainstem, thalamic, and basal ganglia angiographically occult vascular malformations. Neurosurgery 2000;46:260–270, discussion 270– 271 PubMed
89. Flickinger JC, Kondziolka D, Lunsford LD. Risks and benefits of arteriovenous malformation radiosurgery. Tech Neurosurg 2003;9:169–174
90. Firlik AD, Levy EI, Kondziolka D, Yonas H. Staged volume radiosurgery followed by microsurgical resection: a novel treatment for giant cerebral arteriovenous malformations: technical case report. Neurosurgery 1998;43:1223–1228 PubMed
91. Pollock BE, Kline RW, Stafford SL, Foote RL, Schomberg PJ. The rationale and technique of staged-volume arteriovenous malformation radiosurgery. Int J Radiat Oncol Biol Phys 2000;48:817–824 PubMed
92. Batra S, Lin D, Recinos PF, Zhang J, Rigamonti D; Medscape. Cavernous malformations: natural history, diagnosis and treatment. Nat Rev Neurol 2009;5:659–670 PubMed
93. Liscák R, Vladyka V, Simonová G, Vymazal J, Novotny J Jr. Gamma knife surgery of brain cavernous hemangiomas. J Neurosurg 2005;102 (Suppl):207–213 PubMed
94. Aryan HE, Nakaji P, Lu DC, Alksne JF. Multimodality treatment of trigeminal neuralgia: impact of radiosurgery and high resolution magnetic resonance imaging. J Clin Neurosci 2006;13:239–244 PubMed
95. Nurmikko TJ, Eldridge PR. Trigeminal neuralgia—pathophysiology, diagnosis and current treatment. Br J Anaesth 2001;87:117–132 PubMed
96. Pollock BE, Phuong LK, Gorman DA, Foote RL, Stafford SL. Stereotactic radiosurgery for idiopathic trigeminal neuralgia. J Neurosurg 2002;97: 347–353 PubMed
97. Maesawa S, Salame C, Flickinger JC, Pirris S, Kondziolka D, Lunsford LD. Clinical outcomes after stereotactic radiosurgery for idiopathic trigeminal neuralgia. J Neurosurg 2001;94:14–20 PubMed
98. Nicol B, Regine WF, Courtney C, Meigooni A, Sanders M, Young B. Gamma knife radiosurgery using 90 Gy for trigeminal neuralgia. J Neurosurg 2000;93(Suppl 3):152–154 PubMed
99. Rogers CL, Shetter AG, Fiedler JA, Smith KA, Han PP, Speiser BL. Gamma knife radiosurgery for trigeminal neuralgia: the initial experience of The Barrow Neurological Institute. Int J Radiat Oncol Biol Phys 2000; 47:1013–1019 PubMed
100. Kondziolka D, Zorro O, Lobato-Polo J, et al. Gamma Knife stereotactic radiosurgery for idiopathic trigeminal neuralgia. J Neurosurg 2010; 112:758–765 PubMed
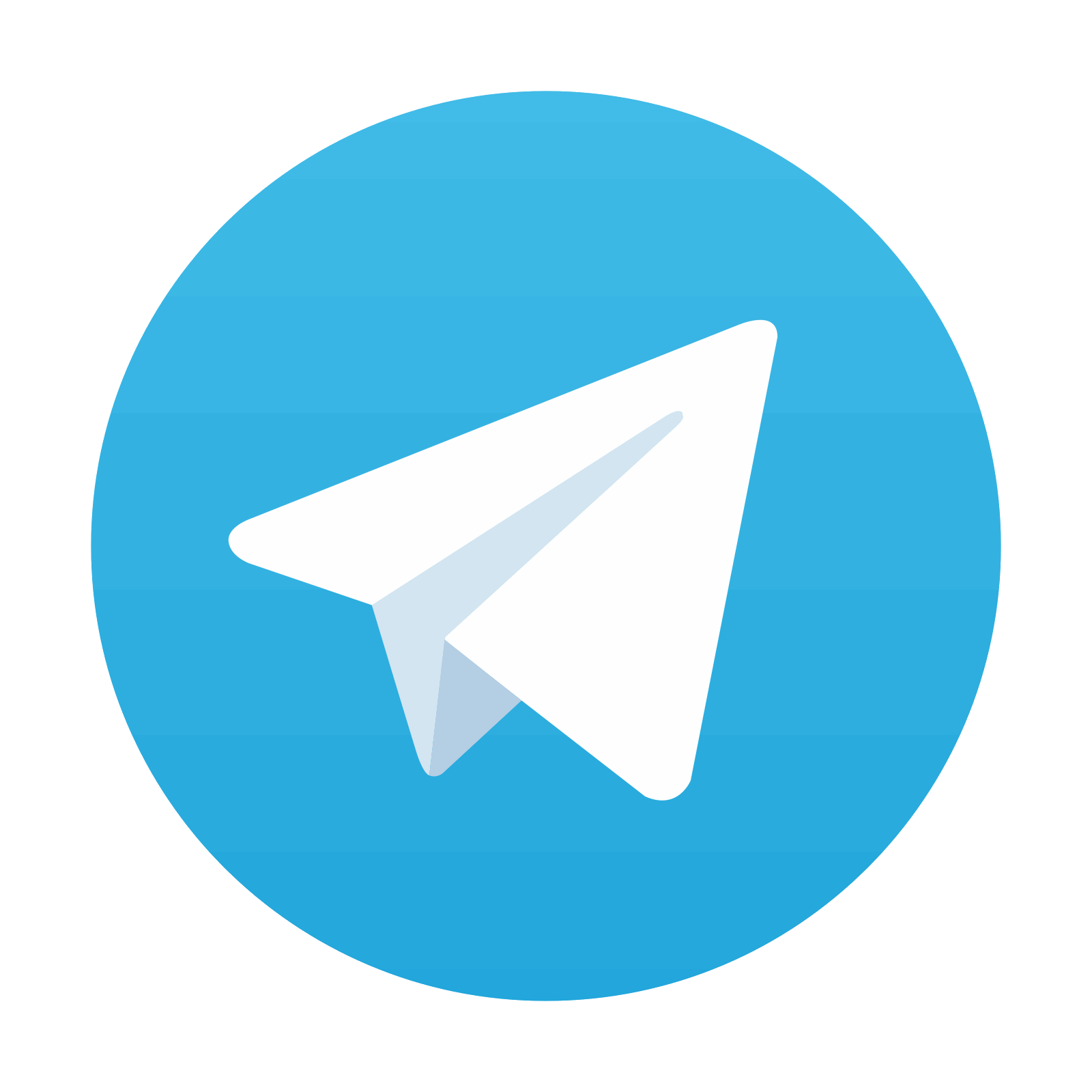
Stay updated, free articles. Join our Telegram channel

Full access? Get Clinical Tree
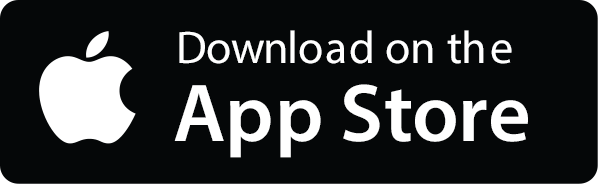
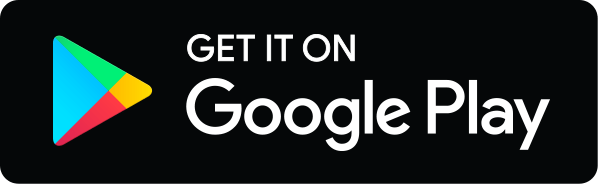