Metastatic Tumors
Enid Choi
Graeme F. Woodworth
Minesh P. Mehta
INTRODUCTION
It is estimated that 13.7 million people living in the United States today are alive with a diagnosis of cancer. The American Cancer Society projects that 1,665,540 new cancer diagnoses will be made in 2014. The American Society of Clinical Oncology predicts that cancer will overtake heart disease as the most common cause of death by 2030, and it is already the leading cause of death in England and in Americans younger than 85 years old. Metastatic disease, that is, cancer that has spread from its site of origin (from the Greek meta, meaning “next,” and stasis, meaning “placement”), is the cause of death in the vast majority of cancer patients. This chapter will focus on these tumors, first discussing them in general and then discussing more specific clinical entities, including brain metastasis, epidural spinal column metastasis, and leptomeningeal metastasis (LM).
METASTATIC TUMORS
EPIDEMIOLOGY
Although the number of new cases of metastasis to the central nervous system diagnosed yearly is estimated at over 200,000, the exact incidence remains unknown. The reason for this is that although cancer as a disease is reportable, sites of metastatic disease are not routinely reported to any registry. Autopsy studies have reported the presence of intracranial metastases in 10% to 30% of all cancer patients (15% to the brain, 5% to the leptomeninges, 5% to the dura) as well as occult LM in an average of 20% of all cancer patients. The incidence of brain metastases has risen steadily over the last few decades, a phenomenon which is likely multifactorial: High-resolution imaging allows brain metastases to be detected more readily, and increasingly effective treatments lead to prolonged survival and thus a longer period during which neural metastases may develop. One conjectured reason for the very high incidence of metastatic disease to the brain is the highly selective permeability of the blood-brain barrier, which allows tumor cells sanctuary in the central nervous system (CNS) both from immune surveillance and the cytotoxic effects of chemotherapy, as most of these agents fail to cross the barrier adequately.
Although the brain parenchyma is the location most likely to be involved (80%) with metastatic disease to the nervous system, other common sites include the epidural space and leptomeninges. Less common sites include the dura, intramedullary spinal cord, optic apparatus, and cranial and peripheral nerves. Data regarding these less common sites are generally limited to case reports and small series, and therefore, this discussion will focus on CNS metastases, primarily of the parenchymal brain.
PATHOBIOLOGY
The molecular processes within cancer cells and their microenvironment that ultimately result in the development of metastatic disease are poorly understood and are the focus of much ongoing research. It is known that the shedding of tumor cells into systemic circulation is an early phenomenon in the natural history of malignancy, but these circulating tumor cells do not always lead to metastasis. It is estimated that less than 0.01% of these cells are able to establish a metastatic lesion at a distant anatomic site. This could well be a consequence of immune surveillance mechanisms. It is also known that certain malignancies have a predilection to metastasize to particular organs. This may be due in part to simple anatomy and physiology but may also be dependent on specific molecular characteristics, including the secretion of specific chemotactic factors by the organ or site involved; neurotropic growth factors in the CNS are thought to play such a role. Another possible factor is the expression of specific surface molecules on tumor cells that allow them to home to certain tissues. Melanoma cells are presumed to possess such molecular features, which allow them to have among the highest probabilities of metastasizing to the brain.
Possible pathways for spread of cancer include hematogenous, lymphatic, intrathecal, and perineural. (Fig. 97.1). Although not technically metastatic, tumors may directly extend into neighboring structures, including the CNS; for example, large tumors of the head and neck may invade the brain or spinal cord. The intravasation of the cancer cell requires multiple signals between that cell and its surrounding microenvironment. Some data suggest that transformation of the malignant cell into a mesenchymal, or connective tissue-like, state is crucial for this, a phenomenon referred to as epithelial-to-mesenchymal transformation, or EMT.
Once intravasated and in circulation, normal anatomic organs may act as a barrier. Regional lymph nodes may trap tumor cells. The hepatocytes of the liver filter the blood as the venous return from abdominal organs passes through it, and the liver is a frequent site of metastatic disease from gastrointestinal primaries. The blood-brain barrier presents another obstacle for circulating tumor cells. Logically, organs with a large volume of blood flow are more prone to metastatic disease, such as the pulmonary capillary bed with its high flow. The CNS and the bones receive approximately 20% of the cardiac output; it is therefore not surprising that the liver, lung, CNS, and bones are the most common sites of metastatic disease.
When cancer cells arrive at a distant site, they must extravasate from circulation and establish at this location, a complex process that requires multiple interactions with the new microenvironment. The nascent metastasis must also be capable of angiogenesis, the formation of new blood vessels, in order to sustain growth larger than approximately 1 mm. Despite all these required steps for the formation of distant metastases, metastatic disease from cancer is an all too real and common phenomenon, indicating that
this orchestrated process of dissociation, intravasation, circulation, avoidance of immune surveillance, extravasation, and metastatic growth is efficiently coordinated by a significant number of malignancies through sophisticated genetic interplay.
this orchestrated process of dissociation, intravasation, circulation, avoidance of immune surveillance, extravasation, and metastatic growth is efficiently coordinated by a significant number of malignancies through sophisticated genetic interplay.
CLINICAL FEATURES AND WORKUP
Metastatic disease to the nervous system may present with or without symptoms, and an appropriate clinical workup is indicated to make the diagnosis. In asymptomatic patients, metastatic disease is often found incidentally during their cancer workup and staging process or while concomitant nonmalignant comorbidities are being investigated. CNS metastases are often diagnosed in the context of a known history of malignancy, but symptomatic CNS metastasis may also be the initial clinical presentation of a previously unidentified malignancy. In this situation, biopsy or resection is required in order to pathologically confirm a diagnosis of cancer and to guide treatment. The most common primary malignancy that presents with metastatic disease in the brain is lung cancer. The most common primary malignancies that presents with metastatic disease to the spine are breast and prostate cancer. In some cases, the primary malignancy responsible for the metastatic disease is never identified despite a complete and thorough evaluation.
History and Physical Exam
The clinical presentation of CNS metastasis is highly dependent on the specific neurologic structure involved and the degree to which it has been compromised. Symptoms may be of gradual or abrupt onset, which provides insight not only to the pathologic process but also prognosis and likelihood of recovery. Clinical workup of a new neurologic deficit must begin with a thorough history and physical exam, with particular focus on the elucidation of any neurologic deficits.
Laboratory Studies
Blood work has limited use in the workup of CNS metastatic disease, but one exception is tumor markers: cancer-specific or cancer-associated proteins that are measurable in the blood or cerebrospinal fluid (CSF). If markers are elevated, especially in the CSF, then systemic metastases, including neurologic, are more likely. The converse statement, however, is not true; a tumor marker value within normal limits does not preclude malignancy or metastatic disease. Despite this limited use, any patient with general neurologic symptoms, such as altered mental status, headache, or seizures, should have basic labs drawn in order to rule out other etiologies such as infection, electrolyte imbalance, toxins, and blood gas abnormalities.
Imaging
Magnetic resonance imaging (MRI), with and without gadolinium contrast, is the gold standard imaging test for suspected CNS cancer. Computed tomography (CT) is used in patients who cannot tolerate MRI and occasionally to further evaluate hemorrhage. Magnetic resonance spectroscopy can suggest whether a lesion is neoplastic, inflammatory, or other. At present, positron emission tomography (PET) with 18F-fluorodeoxyglucose (FDG) has limited use in evaluation of brain cancers due to the high background activity of the normal brain. It is important to note that none of these imaging techniques replaces histology for diagnostic accuracy.
Tissue Diagnosis
In many cases, histologic confirmation of a CNS metastasis is unnecessary, as the likelihood of metastatic disease from a known primary, especially in the setting of systemic disease, outweighs the likelihood of a new primary CNS tumor. This is particularly true if the imaging studies have characteristics consistent with metastatic disease. In other cases, however, the diagnosis of CNS metastasis is unclear from the history, physical exam, laboratory values, and radiographic studies. If the diagnosis of metastatic disease is in question, and if the lesion is surgically accessible with reasonable safety, biopsy should be performed to provide histopathologic diagnosis.
Another important possible method of identifying metastasis to the CNS is sampling of the CSF with a lumbar puncture (LP). LP may also rule in or out other etiologies and clarify the diagnosis of LM (also termed leptomeningeal carcinomatosis, neoplastic meningitis, or carcinomatous meningitis). It will not, however, diagnose parenchymal brain metastases in the absence of CSF involvement. LP is contraindicated due to the risk of brain herniation in cases where increased intracranial pressure (ICP) is suspected.
TREATMENT
Both symptomatic and definitive treatments are important. Symptomatic treatment includes corticosteroids, treatment of seizures, and anticonvulsant prophylaxis. Definitive treatment includes surgery, radiation therapy, and chemotherapy.
Corticosteroids and Antiepileptics
Metastatic disease may cause marked edema due to extravasation of plasma from leaky tumor vasculature. Within the narrow anatomic confines of the CNS, this edema may cause increased ICP and significant compression of the normal neural tissue (Fig. 97.2A). Symptoms of increased ICP include headache and blurred vision. Intravenous corticosteroids, most commonly dexamethasone, should be given immediately to symptomatic patients and often result in rapid improvement in symptoms. One widely used dosing of steroids begins with an intravenous bolus of 10 mg of dexamethasone (although the actual value of the intravenous route over oral as well as the larger 10-mg dose in terms of symptom resolution is unclear), followed by 4 mg given every 6 hours. The dose should be titrated upward or downward as needed. Large doses can cause acute psychiatric symptoms, and chronic administration has myriad toxicities including candidiasis, increased risk of pneumocystis pneumonia, metabolic imbalance, sleep and mood disturbance, muscle atrophy and wasting, weight gain, osteopenia and pathologic fractures, gastrointestinal irritability, and perforation. Steroids should be tapered as rapidly as tolerated once definitive therapy has been initiated.
Symptomatic patients or those with radiographic evidence of significant mass effect should be stabilized with steroids as soon as possible. Steroids have been shown to improve or resolve symptoms in up to two-thirds of patients and may confer a small survival benefit compared to no treatment. Patients who present with seizures should be stabilized with antiepileptic medications, preferably selecting agents that do not induce cytochrome p450 hepatic enzymes, as such induction could interfere with some chemotherapies. Antiepileptics are generally not indicated in patients with no history of seizure, as they have no proven benefit given prophylactically and may have significant adverse effects, including fatigue and decreased cognition; however, studies suggesting
antepileptics have risk outweighing benefit in seizure naive patients were conducted before some of the more modern drugs, such as levetiracetam, were developed, and definitive data are lacking in the modern era [Level 1].1
antepileptics have risk outweighing benefit in seizure naive patients were conducted before some of the more modern drugs, such as levetiracetam, were developed, and definitive data are lacking in the modern era [Level 1].1
Surgery
In general, surgical management is considered for patients who are medically fit, with large tumors (>2 to 3 cm), one or few tumors, and/or a tumor associated with significant brain swelling, mass effect, and neurologic deficits. The benefits of direct tumor removal with surgery include rapid improvement in symptoms and improved local disease control. The decision for or against surgery depends on many factors, including overall clinical performance status; status of systemic disease; and location, size, and number of metastases. Surgery may be followed by radiation or, less commonly, chemotherapy. Risks of surgery include bleeding, infection, CSF leak, and transient or permanent neurologic deficit.
Radiation Therapy
Radiation therapy (RT) may be used in combination with surgery, typically as adjuvant therapy following maximal resection or as primary therapy for unresected disease. Tumor regression following radiation is a gradual process, and the therapeutic effects lack the immediacy of surgical resection, making radiation second-line therapy in situations where immediate decompression is indicated.
For patients with known brain metastases too numerous to target individually, RT is generally delivered to the entire brain (whole brain radiation therapy [WBRT]) with the rationale that the presence of brain metastases indicates that the entire organ has been compromised. The likelihood of other tumor cells with metastatic potential elsewhere within the brain is high, and WBRT not only treats the known lesions but also may prevent the development of macroscopic disease elsewhere in the brain.
WBRT alone may reduce or eliminate symptoms from brain metastases in 60% to 80% of patients and may result in a significant radiographic response in about half of all patients. WBRT is also associated with improved median survival 4 to 6 months as opposed to 1 month without treatment. Side effects include neurocognitive decline, radiation necrosis, and damage related to the specific area of the brain being irradiated.
WBRT is classically given to a total dose of 30 Gy delivered in 10 fractions, although several other fractionation schedules have been shown to have similar efficacy with no significant difference in toxicity (20 Gy in 5 fractions, 30 Gy in 15, 37.5 Gy in 15, 40 Gy in 15, and 40 Gy in 20). Doses higher than this (50 to 54.4 Gy) and hyperfractionation (more and smaller fractions) did not show a survival benefit, and larger fraction sizes were found to be detrimental, with shorter progression-free survival and higher neurologic toxicity [Level 1].2,3,4 Prophylactic cranial irradiation (PCI) is WBRT in the absence of known macrometastatic disease in the brain in order to decrease the likelihood of developing such brain metastasis. PCI is indicated in small cell lung cancer, which has a very high propensity for brain metastasis. Other tumors, particularly classic pediatric brain tumors such as medulloblastoma, have a high incidence of metastatic disease to not only elsewhere in the brain but also the spinal cord. Prophylactic craniospinal irradiation (CSI) is given to the entire CNS axis in these situations.
Stereotactic radiosurgery (SRS) is a more targeted radiation technique in which a much larger dose of radiation is given in a single treatment. The advantages of SRS include shorter treatment time (1 day vs. 2 to 3 weeks) and decreased radiation dose to the surrounding normal brain tissue. SRS may be used safely to treat multiple brain lesions and may also be given in combination with WBRT. In certain situations, the precision of SRS is combined with the delivery of a few large fractions of radiation, as opposed to a single fraction, an approach referred to as stereotactic radiotherapy.
Chemotherapy
Chemotherapy has traditionally had a limited role in the treatment of CNS metastases because the blood-brain and the blood-CSF barriers inhibit the ability of systemic agents to penetrate to the brain and spinal cord. Some agents with good CNS penetration include high-dose intravenous methotrexate and temozolomide. Chemotherapy may also be administered intrathecally, typically via a port placed into the ventricular system, which helps circumvent these natural anatomic barriers, but intrathecal chemotherapy only penetrates into brain parenchyma by a few millimeters at most and is generally ineffective against parenchymal brain metastases. Another limitation to using chemotherapy to treat neurologic metastasis is that the primary malignancy might have previously been treated with the same agent, leading to acquired resistance.
Cancer histologies for which chemotherapy has shown efficacy, albeit modest, in CNS metastases include germ cell tumors (where the effect is dramatic), small cell lung cancer, and breast cancer. Cancers harboring specific driver mutations such as epidermal growth factor receptor (EGFR) mutations in non-small cell lung cancer (NSCLC) may respond well to appropriate targeted therapeutics (e.g., EGFR tyrosine kinase inhibitors), which do at least partially penetrate the blood-brain barrier.
BRAIN METASTASIS
EPIDEMIOLOGY AND CLINICAL MANIFESTATIONS
The most common type of cancer of the brain is metastatic disease; brain metastases outnumber primary brain tumors by a ratio of approximately 9:1. Up to 20% to 40% of all cancer patients will develop lesions in the brain. The brain may be a site of relapse at any time in the clinical course. The median time interval between the development of brain metastasis and the original cancer diagnosis is approximately 1 year, but the range can exceed 10 years. NSCLC is the most common source of brain metastases, likely because it is more common than other cancers. In comparison, a larger proportion of patients with diagnoses such as melanoma, small cell lung cancer, and specific subsets of breast cancer will develop brain metastases, but due to the lower number of patients diagnosed with these diseases, the total incidence from these primary tumors is lower than that from NSCLC.
Symptoms of CNS metastases result from both focal pressure on surrounding tissue, destruction of normal neurons, and global increases in ICP from the metastases or associated peritumoral edema. Presenting symptoms most often include headache (49%), altered mental status (32%), focal weakness (30%), ataxia (21%), and seizures (18%). Diffuse, small, miliary lesions scattered throughout the brain parenchyma are particularly associated with leukemia and small cell lung cancer and may manifest as a generalized encephalopathy (Fig. 97.2B).
Untreated brain metastases generally produce worsening symptoms over days to weeks. In some cases, symptoms are more acute and may mimic ischemic or hemorrhagic stroke. The more abrupt clinical course may particularly be seen with bleeding within the metastasis; hemorrhagic brain metastases are classically associated
with certain histologies, including melanoma, renal carcinoma, choriocarcinoma, NSCLC, and thyroid (Fig. 97.2C).
with certain histologies, including melanoma, renal carcinoma, choriocarcinoma, NSCLC, and thyroid (Fig. 97.2C).
IMAGING
In the setting of a known diagnosis of cancer and new neurologic symptoms, multiple, spheroidal, enhancing brain lesions, mostly at the gray-white junction, with a disproportionate degree of edema on MRI, strongly suggest a diagnosis of brain metastases. Differential diagnosis include primary brain tumor, lymphoma, abscess, other infectious processes, stroke, multifocal demyelination, radiation necrosis, etc. A seminal series of 54 patients with a single brain lesion seen on imaging who underwent resection or biopsy reported pathologic discordance in 11%; in other words, 1 of 10 patients with MRI findings consistent with a single metastatic lesion in the brain might not have a brain metastasis.
Brain metastases may be single or multiple. The classic appearance of metastatic disease in the brain is a round, irregular, ringenhancing lesion at the gray-white matter interface with associated vasogenic edema (Fig. 97.2D). In comparison, high-grade gliomas may appear more infiltrative and irregularly shaped; gliomas are most commonly single and rarely present as multiple distinct lesions. CNS lymphoma tends to appear as periventricular, homogenously enhancing disease. Intracranial abscesses are high on the list of differentials in the setting of immunosuppression. These appear as well-circumscribed, thick-rimmed, ring-enhancing masses with central hypodensity. Stroke, both hemorrhagic and ischemic, may produce both similar symptoms and imaging findings as brain metastases. Multifocal demyelination has been reported after treatment with certain chemotherapy agents (5-fluorouracil and levamisole) and may also mimic metastases in appearance. Radiation necrosis is a known potential complication from RT, particularly SRS, and may also appear as a contrast-enhancing mass (Fig. 97.2E).
About 80% of brain metastases involve the cerebral hemispheres, 10% the cerebellum, and 5% the brain stem, a rate of incidence that appears consistent with the relative volume and percentage of blood flow. A high proportion of brain metastases are seen in the watershed regions. This is most likely due to smaller blood vessels and slower blood flow in these areas. For reasons that are not fully understood, different histologies may preferentially metastasize to different brain locations; lung cancer tends to metastasize to the cerebrum, whereas gastrointestinal malignancies tend to metastasize to the cerebellum.
TREATMENT
Approximately 30% to 50% of patients with metastatic disease to the brain will die from progressive intracranial disease. Therefore, when considering treatment options, one must consider not only the extent of intracranial disease but also overall disease burden, clinical status, and prognosis. To predict survival in the setting of intracranial metastasis, the Radiation Therapy Oncology Group (RTOG) developed a recursive partitioning analysis (RPA) classifier that stratifies patients based on performance status, age, control of primary tumor, and presence of extracranial metastatic disease. The graded prognostic assessment (GPA) index also takes into account the number of brain metastases and is histologyspecific. Based on these categories, predictions may be made regarding overall survival, which may then be used to potentially guide therapy.
Two clinical scenarios merit additional clarification: (1) a single brain lesion, meaning known disease elsewhere with only one lesion in the brain and (2) a solitary brain lesion, meaning the one metastasis to the brain is the only known site of disease. (It should be noted the literature often uses single and solitary interchangeably.) Single or solitary brain lesions generally warrant aggressive management, as this may have a significant impact on survival. A series by Flannery et al., reported that treatment of single, synchronous brain metastases from NSCLC in addition to definitive thoracic treatment improved survival.
After medical stabilization, definitive treatment with surgery, focused radiation with SRS, WBRT, chemotherapy (including targeted agents in highly selected patients), or different combinations of these modalities are considered. The only clinical trials that have ever demonstrated survival advantage from more aggressive therapy are in patients with single (inclusive of solitary) brain metastasis.
Focal Treatment
Brain metastases may be focally treated with either surgery or SRS. To date, there are no completed prospective clinical trials directly comparing surgery with radiosurgery in the treatment of brain metastases, but in retrospective comparisons, outcomes appear generally similar [Level 1].5
SURGERY
Resection of a limited number of brain metastases, usually one, results in improved outcomes, both for locoregional recurrence and overall survival. For patients with solitary or single brain metastases with controlled systemic disease, resection followed by WBRT prolongs survival (median 40 to 50 weeks) and functional independence and improved neurologic function versus WBRT alone. Table 97.1 summarizes the key randomized surgical trials [Level 1].6,7,8 Appropriate patient selection is crucial because resection of single metastases in patients with a greater burden of systemic disease or with poorer performance status generally does not lengthen survival. This is believed to be the reason behind the lack of survival benefit seen in the trial by Mintz et al., as this study included patients with Karnofsky performance status (KPS) of 50, compared to KPS of 70 in the other two trials. The results of this trial were also potentially confounded by a high rate of surgery following disease progression among patients randomized to WBRT alone.
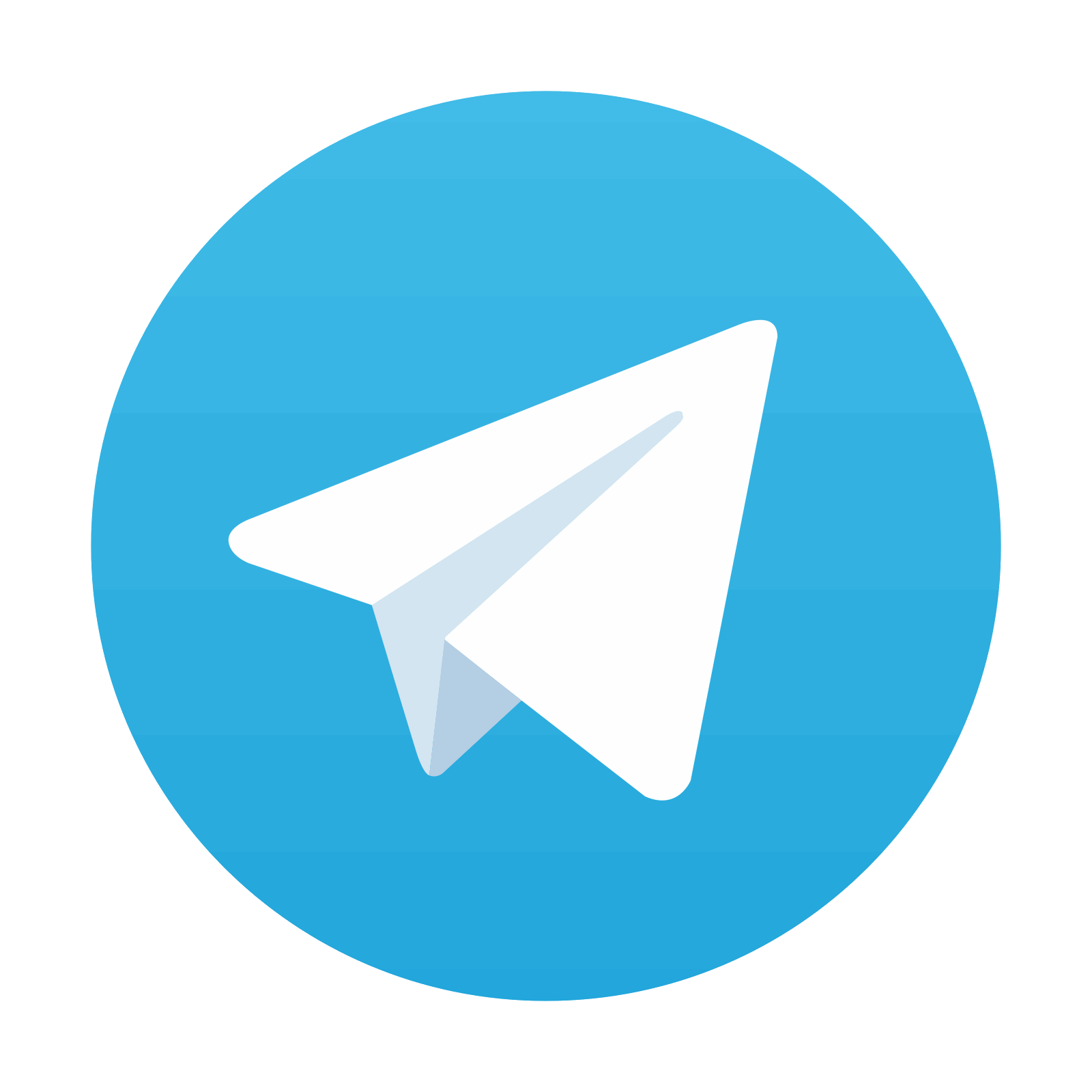
Stay updated, free articles. Join our Telegram channel

Full access? Get Clinical Tree
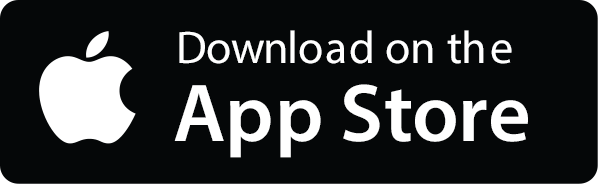
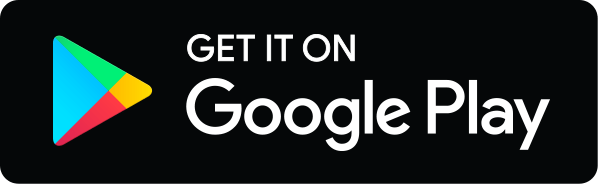
