Progressive hepatolenticular degeneration, or Wilson’s disease (WD), though only a rare cause of movement disorders, occupies an important place in the history of neurology. Over the past century, it has evolved from a confusing, heterogeneous syndrome to a distinct disease entity whose molecular identity is becoming rapidly ascertained. In a 1912 monograph, S. A. Kinnier Wilson, a young neurologist at the National Hospital in Queen Square, elucidated the key features of this previously unrecognized disease (1). His report defined this disorder from extensive analysis of four cases combined with reexamination of six cases previously studied by others. Initially termed “progressive lenticular degeneration” and now synonymous with Wilson’s name, WD represents one of medical science’s successes in deciphering the biochemical and molecular biologic basis for a systemic disease.
WD can take on a puzzling array of clinical findings that can be easily overlooked. In Wilson’s day, several syndromes had been described corresponding to the same disorder he defined. These included entities termed “tetanoid chorea” by Gowers (2), “pseudosclerosis” by Westphal (3) and Strümpell (4), and “Wohl Lues hereditaria tarde” by Homen (5). Wilson’s 1912 report correctly established the relationship between liver involvement and the central nervous system (CNS) disease, and characterized pathologic changes unique to this disorder. He also offered insights into the basis for the distinctive types of clinical impairment. In his monograph, Wilson made no mention of the corneal opacification now recognized as nearly always present in WD with CNS involvement, nor did he have any inkling as to the role of copper despite tissue color changes suggestive of the presence of this metal. Nonetheless, the description of “progressive lenticular degeneration” initiated a flood of clinical and laboratory research, which eventually transformed this illness from a disabling and ultimately fatal condition to a disorder with several treatment options and the potential for presymptomatic diagnosis.
Since WD is inherited as an autosomal recessive disorder, carriers of the gene develop the disease only as homozygotes. As the gene appears to be fully penetrant, all WD homozygotes will develop some form of the disease (and siblings also have a 25% risk). It is extremely unlikely for consecutive generations to be affected, although this has been described (6). Inheritance of the WD gene does not appear to dictate the pattern of predilection for sites of organ involvement, age of onset, or disease severity. Even within the same family, clinical manifestations of WD, including sites of organ involvement and age of onset, can vary greatly (7). The gene for WD has been estimated to be carried in 1 of every 40,000 births. Based on this estimate, the number of homozygotes in the United States would be approximately 5,000 to 6,000 cases, and the carrier frequency may be as great as 1% of the population. Hence, the risk for relatives of an affected patient is 1 in 200 for offspring, 1 in 600 for nieces and nephews, and 1 in 800 for first cousins (8).
In the past decade, linkage analysis studies in affected families have shown that the single disease locus for WD resides on chromosome 13 (9). Most aspects of clinical diversity (i.e., hepatic versus neurologic presentations) probably do not seem to be determined by features of genetic heterogeneity (at least at the level of the gene locus) (8). The discovery of the human gene locus at a single marker interval, 13q14.3 (10), identified a mutation in copper- and membrane-binding ATPase called ATP7B. The protein product of ATP7B is essential for the normal transport and intracellular distribution of copper using an ATP-dependent process (11,12). The regulation of this protein’s expression by copper has recently been characterized with functional gene expression techniques in insects (13). Several ATP7B mutation types (including frame shift, splice site, missense, and nonsense) can lead to WD (14). A further advance in studying the pathophysiology of WD has been aided by the discovery of an animal homologue, a genetic form of hepatitis in the Long–Evans Cinnamon (LEC) rat (15,16). With this model, which is susceptible to systemic toxicity from copper excess resembling that found in WD, various therapeutic interventions such as hepatocyte transplantation can be explored (17).
The pathophysiology of WD has a unitary theme in defective copper metabolism systemically. Copper derived from the diet is normally excreted into the bile (18). All patients with WD are unable to excrete adequate amounts of copper (19–21). Though defect in copper excretion is likely a problem of protein complexing, the exact mechanism has not been determined. WD is not attributable to the defect in the production of ceruloplasmin, a liver-derived copper-binding protein whose serum concentrations are generally (but not always) decreased in WD. As ceruloplasmin is generated by a structural gene on chromosome 3 (and the WD gene resides on chromosome 13), other mechanisms need to be invoked if a defect in ceruloplasmin synthesis is also involved in the pathophysiology of WD (22).
WD provided the first example of a progressive neurologic deterioration that could be explained by a neurotoxic mechanism linked to a biologic defect (23). The disease appears to be solely the outcome from excessive deposition of a dietary mineral, copper, whose presence in trace amounts is necessary for life. Massive amounts of copper are deposited throughout the body at sites where damage to tissues is observed and can be followed with radiolabeled copper studies (24). Correction of copper excretion by means of normalizing liver function can produce full recovery. This has been shown by successful outcomes with autologous hepatic transplantation (25). Removing systemic copper by any means can interrupt progression of WD and often succeeds at reversing neurologic deficits. It is unclear if any additional toxicity is conferred by the increased cerebral uptake of iron that is also demonstrable in WD (26).
| Systemic Manifestations of Wilson’s Disease |
Brain (atrophy, necrosis, gliosis, demyelination, central pontine myelinolysis)
Liver (cirrhosis, acute hepatitis)
Ophthalmologic (corneal Kayser–Fleischer rings; starburst cataract of lens)
Heart (cardiomyopathy)
Kidney (renal lithiasis, hematuria, hypercalcuria, nephrocalcinosis) (28,29)
Anemia (acute hemolytic, thrombocytopenia, Heinz body anemia) (30–32)
Bone and joint disorders (rickets, osteoarthritis, recurrent arthritis, bone fragmentation, chondrocalcinosis, osteoporosis, spinal degeneration, spontaneous fractures, spontaneous rupture of Achilles tendon) (33,34,35–40)
Hypoparathyroidism
Hemosiderosis (41)
Pancreatic exocrine and endocrine impairment (42)
Splenic rupture (43)
Acute rhabdomyolysis (44)
Dermatologic changes, including xerosis, keratosis pilaris, spider angiomas, papulopustular lesions, diffuse hyperpigmentation, sparseness of hair, and localized pigmentation (45)
Sleep disorders, including excessive daytime sleepiness, REM sleep disorders, sleep paralysis, and cataplexy (46)
Infertility or repeated miscarriages (47)
Throughout the body, copper is largely protein-bound. Brain (especially gray matter (27)) and liver, the two organs most susceptible to copper toxicity, possess the greatest avidity for copper binding. The damage copper can produce throughout the body results in a number of clinical syndromes, as listed in Table 24.1. These manifestations of WD can begin monosymptomatically or simultaneously with other clinical features. Although its usual clinical presentations are unmistakable, WD can start in an extremely insidious manner. It has been described with focal, asymmetric features, sometimes without further deficit over several years of follow-up (48,49). Not missing the opportunity for diagnosis when signs and symptoms are mild is one of the greatest challenges posed by this treatable disorder (50–52).
MOTOR FEATURES OF NEUROLOGIC IMPAIRMENT
In addition to a thorough description of hepatolenticular degeneration as a clinical syndrome (1), Wilson gave a detailed analysis of its pathologic imprint on the brain. He further provided a correlation between the motor disorders and the selectivity of its damage to basal ganglia structures. These impairments include dystonic or choreic involuntary movements, tremor, dysarthria, and rigidity. Although the initial presentation of WD can be limited to just one category of movement disorder, the typical case usually involves a combination of each of these motor features. Rarely, other types of movement disorder (such as essential tremor) might coexist in a family with WD (53,54).
Almost half of all WD patients with neurologic disease first experience problems in their second or third decade of life (49,50). For neurologic involvement in WD, the age of presentation tends to be older than with purely hepatic disease. Initial neurologic presentations of WD have been described at less than age 5 years or older than 50 (55–60) or even 70 years (61). Most commonly, juvenile-onset WD tends to present with hepatic damage, whereas older individuals with WD are more likely to have neurologic symptomatology early in the disorder. In children, WD tends to have a more rapid course than in adults (62). Another puzzling aspect of WD is the marked variety in neurologic presentations, even in families. Even with its propensity for asymmetric or focal deficits, virtually all cases of WD have some degree of bilateral pathologic change in the lenticular nuclei. The broad range of neurologic impairments in WD can also be attributed to the sometimes-extensive pathologic change beyond the boundaries of the basal ganglia.
Early diagnosis of WD requires the recognition of potentially subtle initial clinical features. Variable signs and symptoms that are intermittent or hard to categorize can result in delayed diagnosis. It is important to recognize that, in WD, the excess copper load can be quite severe even in patients with only mild neurologic impairments. Also, patients with severe liver damage may be lacking any systemic illness or evidence of CNS involvement. Even certain abrupt systemic manifestations of WD (such as hemolytic anemia or liver failure) can appear with little or no change in neurologic impairment. A sudden or gradual decline in hepatic function caused by WD can, by itself, generate an extrapyramidal movement disorder and encephalopathy apart from the direct damage that copper deposition can inflict on the brain. Pathologic examination can readily distinguish the two disorders. Unlike changes found in WD, hepatic encephalopathy usually is characterized by neuronal damage with a pseudoulegyric pattern but lacking the necrotic changes in the putamen or frontal gyri as found in WD (63) (see below).
Typically, neurologic WD presents insidiously with tremor, dystonia, rigidity, dysarthria, drooling, dysphagia, unsteady gait, and mental deterioration (64). Less commonly, the neurologic syndrome can present more suddenly in association with an irregular fever, emaciation, and a seemingly toxic state. Among the most common initial signs are dysarthria (described by Wilson (65) as “a trifling indistinctness of speech”), clumsiness of the hands, and mental change (“a childishness seen in facile laughter, or irritability, or caprice”). Another series of neurologic WD (44 cases) found 12 presenting predominantly with basal ganglia–related abnormalities, 6 with cerebellar impairment, and 17 with a combination of these (33). Others have made similar observations with the types of neurologic dysfunction (66). The experience of Walshe and Yealland (50) indicated that parkinsonian signs appeared in almost half the patients. Approximately one-quarter of presentations involved a “pseudosclerotic” syndrome, resembling a common pattern of multiple sclerosis involvement by causing cerebellar outflow tremor and speech ataxia. The remainder of patients in the latter study (mostly children) presented with primarily choreic or dystonic features. The disruption of dopaminergic neurotransmission in striatal structures, as demonstrated in neuroimaging studies, is probably responsible for the development of parkinsonian features, dystonia, and dyskinesia in WD (67).
Tremor in one or both hands is probably the most common initial symptom of WD (68,69). Tremor can be unilateral or bilateral, near-constant or paroxysmal, or initiated only during specific motor tasks. Most commonly, the limbs and the head are affected. The tremor in WD can affect limbs at rest, with postural maintenance, or during movement (or any combination of these). Though the action tremor can resemble that observed with cerebellar dysfunction, other signs (such as dysdiadochokinesis, nystagmus, or the Holmes rebound sign) are generally lacking. One characteristic pattern of tremor in WD involves a coarse, irregular, to-and-fro movement elicited by action. When the arms are held forward and flexed horizontally, this maneuver can bring out a proximal component of tremor activity with a “wing-beating” quality. Wing-beating tremor is usually associated with lesions close to the red nucleus that interrupt the striatonigral and dentatorubral pathways (70).
The types of tremor found in parkinsonism and or dystonia may also occur in WD. Less commonly, tremor presents in the trunk or in the tongue (65,71). Chorea and athetosis have been reported to occur in 6% to 16% of patients with neurologic WD, especially in young-onset disease and in combination with other motor signs (72). Those with tremor-predominant disease may have slower progression than those with dystonic-predominant disease. In general, younger patients are more likely to have chorea and dystonia at onset as compared to older patients, who are more likely to have tremor (72). Motor dysfunction in the territory of the bulbar musculature is prominent in WD and in one series (73) was more common than tremor as an initial feature. Drooling, dysarthria, cranial dystonia, and pharyngeal dysmotility (74) all can be observed. Other types of motor impairment can involve the cranial region, especially when dystonic features develop elsewhere in the body (73). Among these are facial grimacing, blepharospasm, and tongue dyskinesia (75). A fixed, “sardonic” smile with retracted lips and opened jaw was illustrated in Wilson’s original report (64) and is still a highly typical feature. This facial dystonia can often progress to impair closure of the mouth. Spasmodic movements of dystonia can also occur in cranial or cervical musculature. The varieties of dystonia in WD include generalized, segmental, or multifocal patterns, such as bilateral foot dystonia (76). The presence of dystonia often correlates with changes in the putamen seen on magnetic resonance imaging (MRI) (72).
Virtually all untreated patients are affected by progressive disturbance of speech. Dysarthria is the most common presenting symptom of WD, often mixed with spastic, hypokinetic, and dystonic qualities. The disturbance of speech is due to impaired motor control of lip, tongue, and pharyngeal muscles. Wilson describes dysarthria in this disorder as “reproduc[ing] the slurring but not the staccato element of multiple sclerosis” and can progress to complete anarthria. Lesser degrees of speech impairment include indistinct articulation, loss of consonant production, a “whispering dysphonia” (77), and marked slowing in the cadence of speech (62,78). Walshe and Yealland have commented on a characteristic inspiratory laugh in WD which “once heard, is never forgotten” (50).
In early WD, there can be other types of motor impairment, some of which can be difficult to categorize. Patients tend to become clumsy and slow at everyday tasks. Handwriting and other limb movements may reflect loss of dexterity, as evidenced by diminished ability to tap fingers rapidly (79). Handwriting changes in WD may resemble the micrographic features typical of Parkinson’s disease. Trunk titubation, a change of posture, or gait disturbance is commonly seen even in mild cases. Muscle cramps may be experienced (79). WD can also present with a typical unilateral or bilateral parkinsonian syndrome. Sometimes, clinical features initially observed do not correspond to the basal ganglia involvement, which is nearly always found in imaging the brain (see below). For example, ataxia has been described as the only clinical manifestation in a chronic case of WD (58). Sometimes, early manifestation of WD is an unusual task-specific difficulty, such as inability to maintain a particular head posture or to protrude the tongue (62). Sustained, abnormal postures characteristic of dystonia are especially common and, in addition to the bulbar region, can affect the trunk and limbs. The intensity of rigid dystonic posturing can be so great as to require tendon release for relieving disability.
The basis for clinical heterogeneity in presenting features is not understood (80), although it has long been recognized that some patients develop predominantly dystonic or parkinsonian features, and others are affected more with bulbar dysfunction and tremor. For this reason, WD should be highly suspected with respect to any number of categories of movement disorder. Spasticity usually does not develop, although patients can exhibit hyperreflexia and Babinski signs. An early manifestation of WD can be pseudobulbar palsy with involuntary bouts of crying or laughter (81). The latter problems, when advanced, can be highly disabling. Other types of movement disorders, such as tics and myoclonus, are not generally observed. Unusual stereotyped movements and tics have been reported (70), and in one case of WD that presented as a tremor disorder, there was also episodic truncal myoclonus, priapism, and seminal ejaculation that occurred several times per day (82).
SEIZURES
In Wilson’s 1912 monograph, instances of epilepsy are described. Though seizures are not a common occurrence in WD, some reports have documented either focal or major motor seizures at any stage of the disorder (83,84). Seizures have been described in cases lacking other evidence for CNS involvement, and are more common in juvenile WD patients. One large series of cases found the prevalence of seizures in a WD group to be 6.2% (85). The latter prevalence corresponds to an approximately tenfold increase over seizure occurrence in the general population. In some reports, convulsions begin to occur at initiation of decoppering treatment. Seizures sometimes can be confused with other paroxysmal movements, such as tremors or dystonia, which sometimes occur as intermittent events in WD.
BEHAVIORAL AND MENTAL STATUS FEATURES
A spectrum of changes in behavior and mental status can occur in WD. Among these are anxiety, mood alterations, impulsivity, as well as other psychiatric disorders. Wilson’s original case reports included the description of “emotionalism,” though he regarded such behavioral features to be transient (1). However, subsequent study of WD has shown how commonly this disorder produces an affliction of the mind. Such impairment can be evident in disturbances of school performance, personality, or behavior. Prominent abnormalities on the Minnesota Multiphasic Personality Inventory and other evaluations of personality traits may be found (86). Other early signs of WD include a decline in memory function or cognitive abilities (87–89). Cognitive impairment when present usually falls in to two categories, a frontal lobe syndrome (characterized by impulsivity, impaired social judgment, promiscuity, decreased attention, executive dysfunction, poor planning, and decision-making) and subcortical dementia (characterized by bradyphrenia, memory loss, and executive dysfunction without cortical signs) (70). Some aspects of neuropsychological performance tend not to be impaired, such as rate of information processing (90). Patients with WD have been described to exhibit schizophreniform or paranoid features, sometimes with hallucinations or delusional thinking (91,92). Psychiatric disorders in WD without psychotic manifestations include depression or other mood disorders, lethargy, nightmares, disinhibited behavior (such as inappropriate laughter), or loss of insight (51,89,93).
As many as 50% of patients with WD developed some form of psychiatric disturbance as an initial feature (70,94,95). Other studies have estimated an even greater incidence of psychiatric disturbance, such as moderate to severe depressive traits (96,97). Prior to a confirmed diagnosis of WD, another series found that 17 of 34 patients had received psychiatric treatment for various disorders, including schizophrenia, depression, and anxiety (98). Depression can be a presenting feature (99). With other types of neurologic involvement, the likelihood of a behavioral disorder is much increased. Among these are behaviors such as impulsiveness, self-injuriousness, suicidal ideation, aggressiveness, sexual preoccupations, and childishness (91). Other behavioral disturbances include irritability and a low threshold for anger. A decline in schoolwork or work performance is almost always evident (97). Antisocial or criminal behavior may occur (100). Case reports of WD appearing with anorexia nervosa (101), hypersomnia (102), or catatonia (103) have been described. When untreated, patients with WD can experience progressive decline in cognitive abilities as a consequence of neuropathologic changes throughout the brain or as a secondary consequence of liver failure. Dementia from WD is usually irreversible. A striking finding in one study was the lack of correlation between the extent of copper toxicity and the features of neuropsychological impairments in both symptomatic and asymptomatic WD patients (98).
OPHTHALMOLOGIC FEATURES
Opaque or mottled bands of pigmentation in the peripheral cornea are characteristic of WD when it involves the CNS (104,105). Although this feature escaped recognition by Wilson, these corneal rings were discovered prior to description of hepatolenticular degeneration. Independent reports by Kayser (106) and by Fleischer (107) are the basis for the eponym now applied to these distinctive changes in the cornea, caused by deposition of copper. Eventually, clinicians came to recognize the strong connection between the corneal pigmentation and the progressive neurologic and hepatic disorder described by Wilson.
Kayser–Fleischer rings have a brownish or greenish tint, and generally arise in the periphery of the cornea. Though usually they appear uniformly concentric around the cornea, these rings can be especially prominent at the upper pole (and can require lifting of the eyelid for recognition). Rarely, Kayser–Fleischer rings can be unilateral (58,108). Kayser–Fleischer rings can often be identified without magnification or special illumination, especially in individuals with blue irises. However, a definitive analysis for the presence or absence of these rings requires a careful ophthalmologic slit-lamp examination. With this magnified view of the eye, another characteristic finding in WD can be discerned, if present. The “sunflower” cataract is an opacification in the lens also caused by copper (109). Though a less common finding in WD than the Kayser–Fleischer ring (110), sunflower cataracts have the same significance. Using Scheimpflug photography, the clinical course of corneal and lens opacification can be followed (111).
Copper-containing granules compose the Kayser–Fleischer ring, which develops primarily in Descemet’s membrane of the cornea. These rings and the sunflower cataract arise simultaneously with the onset of neurologic manifestations of WD (110). The intensity of the rings tends to decrease with institution of decoppering therapy (112) or after liver transplantation (113), occasionally disappearing from view. The majority of CNS WD cases demonstrate Kayser–Fleischer rings. When hepatic disease alone is present, Kayser–Fleischer rings are only present in approximately half of diagnosed cases and are lacking in up to 5% of patients with neurologic manifestations (47). In exceptional circumstances, they can be absent in otherwise-typical systemic manifestations of WD (50,57,114–117). One report described Kayser–Fleischer rings developing only after the start of penicillamine in a subject whose clinical picture was otherwise highly typical of WD (118). Other disorders rarely give rise to corneal deposits very similar in appearance to Kayser–Fleischer rings (119). These conditions include cirrhosis and other forms of biliary obstruction (120).
In WD, the eye may be affected in other ways. As an early feature of the disorder, reading may be impaired (121), possibly due to gaze distractibility or difficulty in fixation (64,122). Among the types of abnormal eye movements are a defect of supranuclear accommodation (123), a disturbance of smooth pursuit, nystagmus, episodic diplopia, and impaired convergence (49,50). Apraxia of eyelid opening (124) and slowed saccadic pursuit (125) may occur. Presentations of neurologic WD with oculogyric crisis (126) and with progressive visual loss (127) have been described.
NEUROPATHOLOGY OF WD
Much of Wilson’s 1912 monograph on hepatolenticular degeneration was devoted to detailed pathologic analysis of the distinctive changes produced in the brain. While the distribution and severity of histologic changes can differ among cases, pathologic involvement is usually most extensive in the basal ganglia, where there can be cystic or cavitary degeneration. The massive necrosis that can be found in the gross pathology of the cut brain (48,63,128) explains why neurologic impairments are often irreversible. As is evident from neuroimaging studies, the lenticular nuclei are the major targets of atrophic changes. The symmetric shrinkage of the putamen is sometimes the consequence of necrotic changes that tend to be especially prominent laterally. The entire putamen can be shrunken with cavitary necrosis and a cystic or granular appearance. Tissues in the striatum can take on a brown, red, or yellow color, though not the greenish hue sometimes seen in corneas. The pallidum may also exhibit discoloration, but these structures tend not to undergo degeneration. Flattening of the caudate nucleus may occur, though more atrophy in the putamen is usually found. Cystic or cavitary necrosis can occur beyond the lenticular nuclei in claustrum, extreme capsule, thalamus, subthalamic nucleus, and red nucleus (42,48,129). About 10% of cases have atrophic or degenerative changes beyond the lenticular nuclei (128).
Elsewhere in the brain, WD can be associated with dilation of the lateral ventricles and generalized brain atrophy. Rarely, the major brunt of pathologic change is found in cerebral cortical gray and white matter (130–133). Degenerative changes have been described to be most prominent in the superior and middle frontal gyri. Similar degrees of damage can extend as far back as the temporal and parietal cortex. In some cases of WD, damage to myelin has been the predominant pathologic picture. Sometimes, the loss of white matter is so extensive as to suggest the presence of demyelinating disease (130,132,133). Another picture of the CNS pathology of WD involves atrophy in the brain stem and cerebellar folia (134). Atrophy can show a predilection for the superior vermis and the dentate nucleus (135). White matter lesions with the appearance of central pontine myelinolysis have been described in otherwise-typical cases of WD (63,136–138), including development after liver transplantation (139).
On microscopy, pathologic change in WD tends to be more widespread than might be suspected from inspection of the cut brain. In particular, deeper layers of cerebral cortex and adjacent white matter are often the most affected regions. Prominent changes include capillary endothelial swelling and proliferation of glia (especially those of Alzheimer type II) (89,128). Gliosis is most abundant in putamen and can also be present in globus pallidus and caudate nucleus whether or not cavitary or necrotic changes are present. Most cases of severe WD show extensive evidence of damage to myelinated fibers in association with Alzheimer type II gliosis extending diffusely throughout cerebral white matter. A variety of degenerative changes in glia have been described (128), including spongy changes of white matter.
Though a distinctive pathologic change, Alzheimer type II glia are not specific to WD. When observed in other forms of chronic hepatic disease in association with encephalopathy, they may not be as prominently configured in the lenticular nuclei as in WD. Another pathologic finding in most cases of WD is the Opalski cell, which appears to be derived from glia (140). Opalski cells are large, rounded cells with periodic acid–Schiff–positive staining for cytoplasmic granules and an irregularly shaped nucleus. The most common locations for Opalski cells are the cerebral cortex cells and the boundaries of lenticular nuclei cavity. When they are found in this configuration together with Alzheimer type II glia, the pathologic diagnosis of WD is almost certain. Alzheimer type I cells can also be found in WD. In one study, their presence was highly correlated to the extent of reactive astrogliosis and inversely proportional to the severity of Alzheimer type II changes (141). Opalski cells and Alzheimer I and II glia may be involved in a copper detoxification process, since they express the copper-binding protein metallothionein (140).
Other types of neuronal damage may be found throughout the brain, especially for larger neurons. The cerebellar pathology of WD most often consists of prominent damage to white matter surrounding the dentate nuclei and extensive (but nonspecific) loss of Purkinje cells (89). Two reports of cerebral cortex electron microscopy in WD have been published (142,143). In addition to typical degenerative changes in neurons, myelin, glia, and axons, Hirano bodies and protoplasmic astrocytes (probably Alzheimer type II) were also found. In cortical neurons, spheroid bodies were prominent. Similar changes have appeared in the lower brain stem sensory nuclei in WD (144). Activated microglia can be found in WD, with ultrastructural changes demonstrating a number of patterns, including transitional forms between rod, ramified, and amoeboid microglial (145).
The impact of decoppering treatment was analyzed in the brains of 11 WD subjects treated chronically with penicillamine (138). For five of the patients, neurologic features of WD had fully resolved, and the other patients achieved marked clinical improvement. Despite continued copper chelation therapy, brain copper content was greatly increased over control values for eight of the nine brains studied. Furthermore, prominent neuropathologic changes typical of WD were evident in each of the penicillamine-treated patients despite their good neurologic outcomes. These results indicate that chelation therapy with penicillamine does not normalize brain copper content or avert either the gross or microscopic pathology associated with CNS WD. The severity of neuropathologic change and regional cerebral copper content was only weakly correlated.
NEUROPHYSIOLOGIC FINDINGS
Disruption of central conduction pathways can provide a sensitive clue to the impact of WD on the CNS. In general, this disorder does not have pathognomonic findings on any form of neurophysiologic testing, though the results of testing for evoked responses can be useful in the workup of WD (146). Usually, the electroencephalogram has not been informative. Generalized slowing and focal abnormalities may be found (147). Most cases of WD studied by electroencephalographic spectral analysis and topographic mapping have shown slowing or epileptiform activity (148). One study of evoked potentials (EPs) revealed that most WD patients with neurologic involvement had one or more findings of a prolonged EP, as did almost half of those lacking other evidence for CNS involvement (149). Delayed conduction of sensory EPs can show a variety of patterns (147). Whether or not clinical signs are present, patients with WD can have abnormal visual EPs, especially with respect to the P100 waveforms (150–152). Studies of brain stem auditory EPs have shown no abnormality (151) or else prolongation (152). A study comparing all three types of EPs found that those of brain stem auditory and somatosensory pathways were more likely to be delayed than those from the visual pathways (153). In the latter study, prolongation of an EP was determined in all severely affected patients and almost all of those moderately affected by neurologic WD. An abnormal EP was also found in more than half of those judged to be mildly affected and in 4 of 24 lacking any other clinical evidence for CNS involvement. Hence, EP can be highly sensitive to WD in the brain or spinal cord. Brain stem auditory and somatosensory EPs may also show changes in response to therapy for WD (149). Abnormalities of pattern-reversal visual evoked responses and flash electroretinograms can undergo improvement with treatment of WD (154).
Another approach that has been used for studying damage to central conduction pathways has been the study of motor EPs following magnetic stimulation of the motor cortex and the spinal roots (155). Subjects with neurologic WD, whether with demyelination or extrapyramidal features, have shown diminished or absent cortically evoked motor responses (156–158). In one reported case of WD, abnormal electromyographic responses evoked by transcranial magnetic brain stimulation were the only strong abnormal neurophysiologic finding (159). These magnetically-elicited motor responses became normal after a course of decoppering therapy.
DIAGNOSTIC TESTING FOR WD
The initial hope that the deciphering of the genetic abnormality in WD would quickly lead to a simple diagnostic test has been dashed (or at least delayed) by the subsequent identification of what is now greater than 500 distinct mutations in the ATP7B gene (160,161). Not all mutations are known to cause WD. Some populations have single predominant mutations (e.g., Sardinian, Icelandic, Korean, Japanese, Taiwanese, Spanish, and Canary Islands) and genetic testing may be more beneficial in these groups. However, testing for WD gene mutations is often impractical because of accessibility and high cost. The most common ATP7B mutation found in patients of European origin is the histidine-to-glutamate substitution at amino acid 1069 (H1069Q). The H1069Q mutation is found in 37% to 63% of Caucasian populations studied. Some studies have found that the H1069Q mutation is associated with late-onset and neurologic disease, though this notion is not universally accepted. R778L is a common mutation in Chinese patients, found in 34% to 38%. Many if not most patients with WD are compound heterozygotes, having two different mutations on the ATP7B gene (162).
The clinician must still rely on a battery of tests to make the diagnosis of WD, the components of the battery varying with disease stage. Clinical suspicion of this disorder is always the first step, regardless of available laboratory tests. The protean nature of WD’s clinical presentations always makes its diagnosis a challenge. This situation is underscored by Prashnanth and colleagues (163), who reported that diagnostic errors were made by referring physicians in 192 of 307 patients found to have WD. Furthermore, a mean delay in diagnosis of 2 years ensued because of these errors. In light of the pleomorphic clinical character of WD, the diagnosis should be considered in any individual presenting with unexplained hepatic, psychiatric, or neurologic dysfunction—especially if the neurologic dysfunction involves basal ganglia or cerebellar function. While this is especially true for younger individuals, the fact that WD has had an initial diagnosis in some individuals over age 70 (61) means that it should be considered and excluded even in middle-aged and elderly persons with appropriate symptoms. In patients who have a very good recovery (freedom from neurologic signs and symptoms), approximately one-third had therapy initiated in <1 month. In those diagnosed in less than one month, only 12% had a poor outcome versus those diagnosed at >18 months, and 38% had major disability (70). All first-degree relatives should be screened as well (47).
LIVER BIOPSY FOR HEPATIC COPPER CONTENT
Liver biopsy is the most sensitive and accurate test for the diagnosis of WD. Hepatic copper content is significantly elevated in virtually all individuals with WD. Even asymptomatic, or presymptomatic, individuals will have marked elevations of hepatic copper. In WD, the major elevation of hepatic copper generally exceeds 250 μg per gram of dry tissue. However, liver biopsy is not without its diagnostic pitfalls with regard to WD. Intrahepatic copper accumulation, although present, may be undetectable by histochemical staining in more than half of patients in the very early stages of WD because the copper is diffusely distributed in the cytoplasm (164,165). Even when liver involvement is more advanced, tissue copper deposition can be unevenly distributed and actually missed if the biopsy specimen is small. Specimens of 1 to 2 cm should be obtained (47). Thus, a negative copper staining result, by itself, does not exclude the diagnosis of WD (165). Because of this, some investigators propose that double-sample biopsies be performed (166).
Although elevated hepatic copper content is a sensitive diagnostic finding in WD, there also may be alternative explanations for its presence. Obstructive liver diseases, such as primary biliary cirrhosis, biliary atresia, extrahepatic biliary obstruction, primary sclerosing cholangitis, intrahepatic cholestasis of childhood, Indian childhood cirrhosis, and even chronic active hepatitis, also result in elevated hepatic copper (168–172). Copper content that is lower than expected (but still elevated beyond the threshold of 100 μg/g) may be found in WD if the biopsy is obtained when copper is being liberated from the liver into the systemic circulation (89).
Since it is an invasive procedure, liver biopsy is associated with a small morbidity. Therefore, it should be reserved for those situations in which other noninvasive studies do not provide the answer. Liver biopsy is the diagnostic test of choice for an individual presenting solely with hepatic dysfunction, since at this stage the copper load may not have been released from the liver into the general circulation. As mentioned above, clinicians should always keep in mind that a single determination of hepatic copper content might be misleading in WD (173). Hepatic copper levels of <250 μg/g of dry tissue are generally in the normal range, though this number has undergone some criticism for being too high. In one report, lowering the threshold from 250 μg/g dry weight to 75 μg/g dry weight improved diagnostic sensitivity from 83.3% to 96.5%, while diagnostic specificity remained acceptable (95.4% versus 98.6%) (174).
SERUM CERULOPLASMIN
Ceruloplasmin originates as an inactive, non–copper-containing form, called apoceruloplasmin. One of the functions of ATP7B protein is to add seven copper atoms to the apoceruloplasmin, producing holoceruloplasmin, which is the functionally active form of ceruloplasmin (175). Routine measurement of ceruloplasmin usually involves an immunologic assay, which measures total ceruloplasmin (apoceruloplasmin and holoceruloplasmin). Some investigators have suggested that enzymatic assay of ceruloplasmin oxidase activity, which measures only holoceruloplasmin, is a more accurate method of assessment and should be preferentially employed (175,176). Ceruloplasmin, an acute-phase reactant that is secreted from the liver, carries six copper atoms per molecule and accounts for 90% of circulating copper. Serum ceruloplasmin concentration of <200 mg/L (<20 mg/dL) has been consistent with the diagnosis of WD and is diagnostic if Kayser–Fleischer rings are present. Serum non–ceruloplasmin-bound copper concentration is elevated above 25 μg/dL in most untreated patients (normal <15 μg/dL). Measuring the nonceruloplasmin level is problematic for diagnosis because it relies on accuracy of both the ceruloplasmin and serum copper. Non–ceruloplasmin-bound copper is sometimes used for monitoring treatment (162).
Serum ceruloplasmin determination, even when immunologically measured, is a simple and useful (though insufficient) screening test for WD. Ceruloplasmin concentrations are reduced in the majority of individuals with WD, though not in all. For 5% to 15% of WD cases, serum ceruloplasmin concentrations may be in a low-normal or only slightly subnormal range (89,177). It also is helpful to remember that because ceruloplasmin is an acute-phase reactant, it is transiently elevated during pregnancy, during estrogen administration, or during infectious or inflammatory disorders (178). In such circumstances, the diagnosis of WD may be obscured by a normal serum ceruloplasmin concentration (179). Ceruloplasmin levels also may be in the normal range in individuals with WD presenting with fulminant hepatic failure, although 24-hour urinary copper levels will be extremely high (180).
Furthermore, ceruloplasmin deficiency is not unique to WD and can be found in a variety of other disorders, including Menkes’s disease, protein-losing enteropathy, nephrotic syndrome, sprue, and other situations in which protein and total calorie intake are insufficient (178,181
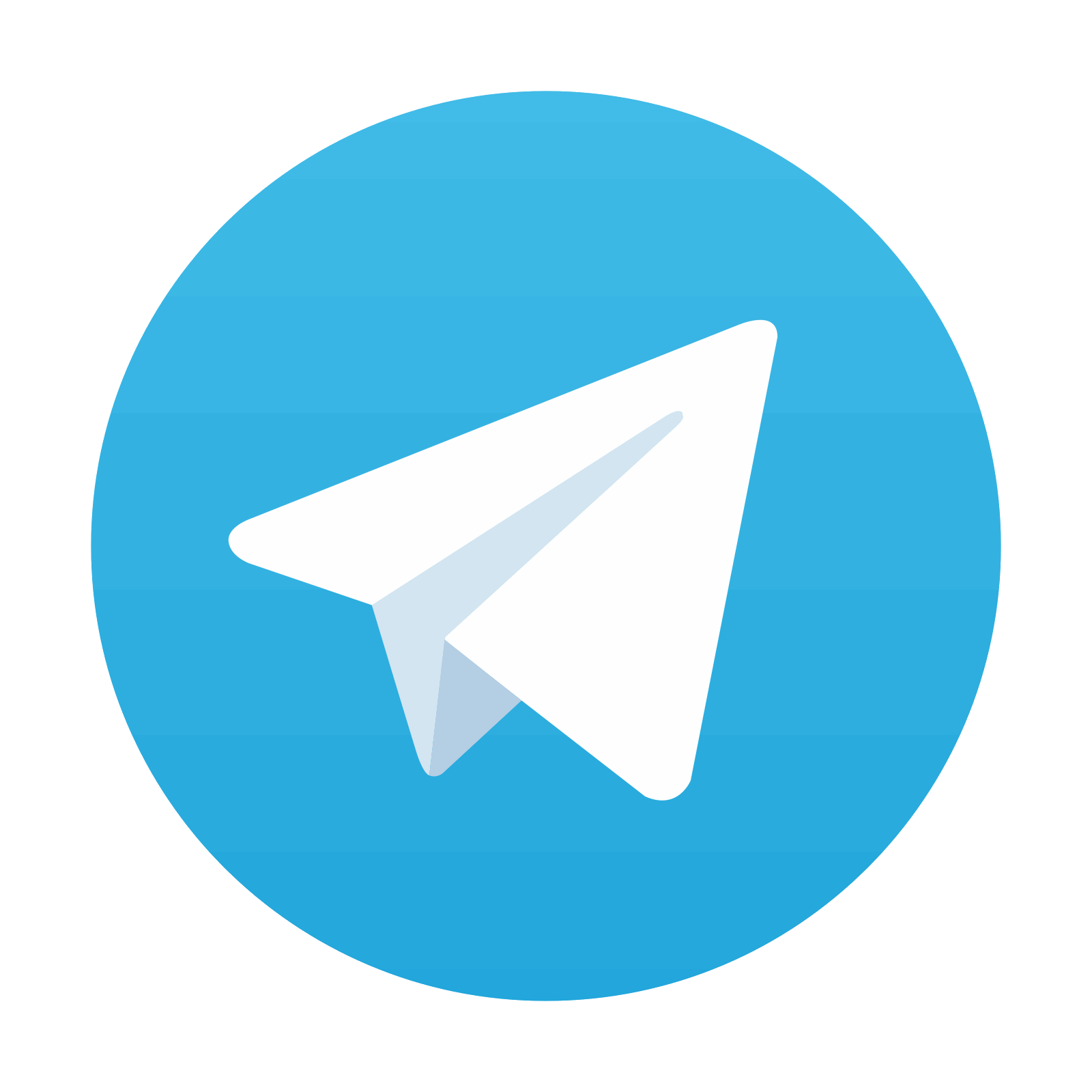
Stay updated, free articles. Join our Telegram channel

Full access? Get Clinical Tree
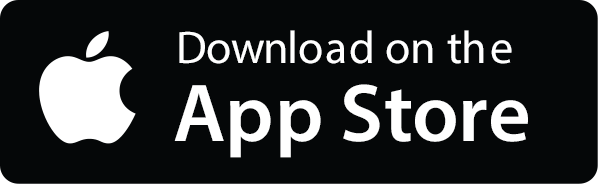
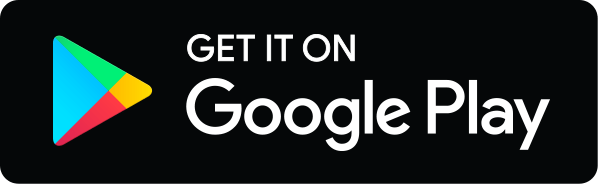