Pathophysiology of Spinal Cord Injury
Timothy B. Himes
Spinal cord injury is not one event, but a sequence of interrelated processes that can ultimately lead to destruction of neural tissue and, as a consequence, a dramatic loss of locomotor and sensory function. Understanding these processes and their chronology starting immediately after trauma and continuing for the weeks, months, and even years afterward is vital to the development of a comprehensive repair strategy for spinal cord injury (SCI). Unlike the peripheral nervous system (PNS), where injured axons can regenerate their damaged axons and reestablish functional connections with peripheral targets, regeneration in the central nervous system (CNS) and spinal cord is extremely limited. Neurons that die as a result of SCI are not replaced by proliferation from stem cells, and the severed axons of surviving neurons cannot reconstruct damaged axonal pathways. However, most human spinal cord injuries, even those that are physiologically complete, are anatomically incomplete and spare both intraspinal and peripheral pathways. Limited but substantial reorganization of unlesioned circuits has been proposed to contribute to the spontaneous recovery of sensory and motor function often seen following SCI. Injured spinal cord neurons do have the capacity to regenerate, however, and for almost 50 years it has been known that injured axons can send out regenerative sprouts in the acute phase following injury (1) and that axonal growth can be extensive if injured axons are provided with a permissive environment (2). Unfortunately the regenerative response collapses before functional benefits are realized. Successful spinal cord repair will ultimately involve several factors, not only restoration of neural cells and circuits lost as a direct consequence of SCI, but also management of factors within the CNS that inhibit successful regeneration.
Embryology, Anatomy, Histology, and Function of Normal Spinal Cord
Embryology
The spinal cord is a roughly cylindrical structure located in the spinal canal of the vertebral column. In humans the spinal cord is about 42 to 45 cm long and 1 cm in diameter. This bilaterally symmetrical structure extends from the medulla caudally, ending, on average, at the level of the disc between the first and second lumbar vertebrae (Fig. 1-1). The spinal cord is conical in shape at its caudal end, a region known as the conus medullaris. From this tapered cone a thin filament, the filum terminale, continues caudally. At approximately the second sacral segment the filum terminale alone becomes ensheathed in dura and continues as the coccygeal ligament to attach to the dorsum of the coccyx. Until the third month of fetal development the spinal cord extends the entire length of the spinal canal. After the third fetal month the lengthening rate of the spinal column is greater than the spinal cord such that in the adult the spinal cord occupies only the rostral two thirds of the spinal canal. It is therefore necessary for the lumbar and sacral nerve roots to descend within the spinal canal a considerable distance in order to exit from the appropriate intervertebral foramina. The filum terminale, surrounded by these lumbosacral nerve roots, comprises a structure known as the cauda equina, which extends in the spinal canal from the second lumbar to the second sacral vertebral segment. This region is also known as the lumbar cistern since there is sufficient subarachnoid space for the accumulation of cerebrospinal fluid (CSF). The withdrawal of a CSF sample by lumbar puncture between the third and fourth lumbar vertebrae can be accomplished in the lumbar cistern with minimal risk of damage to neural tissue, due to the absence of the spinal cord in this region. Covering the spinal cord are the same layers of meninges (pia mater, arachnoid, and dura mater) as those that cover the brain. The spinal cord is suspended in CSF within the subarachnoid space and held in place by a series of denticulate ligaments. The denticulate (dentate) ligaments are extensions of the pia mater that connect the spinal cord to the dura mater laterally. They are located midway between the dorsal and ventral roots and extend from the foramen magnum to below the exit of the first lumbar spinal nerve (Fig. 1-2).
Although the spinal cord grossly appears uniform and symmetrical on the exterior, it is anatomically arranged in segments with paired spinal nerves exiting into the body at each of 31 levels (8 cervical [C], 12 thoracic [T], 5 lumbar [L], 5 sacral [S], and 1 coccygeal) via the intervertebral foramina (Fig. 1-1). In the cervical spinal cord the nerves exit through the intervertebral foramina just rostral to the vertebra of the same name, but since there are eight cervical nerve roots and only seven cervical vertebrae, the eighth cervical spinal nerve exits through the intervertebral foramina just rostral to the first thoracic vertebra. The thoracic, lumbar, and sacral spinal nerves exit through the intervertebral foramina just caudal to the vertebra of the same name. The spinal nerve is composed of a dorsal root of afferent fibers and a ventral root containing efferent fibers. The dorsal and ventral roots are within the dural sheath of the spinal cord until they reach the intervertebral foramina where the dorsal root ganglion is located. An anatomical variation seen in humans is that the C1 dorsal root ganglia and root are missing in almost 50% of the cases with only a C1 ventral root being present. Each spinal nerve is related to a specific somite during development such that in the adult spinal cord segments form a systematic sensory relationship to areas of skin, muscle, and bone. This unit is known as a dermatome, and the understanding of the segmental
innervation of muscles and cutaneous regions is an important clinical tool for diagnosing the site of damage to the spinal cord (Fig. 1-3). Axons outside the spinal cord, in either the dorsal or ventral roots, are no longer associated with processes of CNS glia (astrocytes, oligodendrocytes), but are now nurtured, supported, and myelinated by the Schwann cells of the PNS.
innervation of muscles and cutaneous regions is an important clinical tool for diagnosing the site of damage to the spinal cord (Fig. 1-3). Axons outside the spinal cord, in either the dorsal or ventral roots, are no longer associated with processes of CNS glia (astrocytes, oligodendrocytes), but are now nurtured, supported, and myelinated by the Schwann cells of the PNS.
Gray Matter and Neuronal Pathways
A cross section through the spinal cord shows its organization generally to be opposite that of the brain; with a butterfly-shaped gray matter core of neurons and glia surrounded by myelinated axon fiber bundles and tracts in the outer white matter (Fig. 1-4). Rexed (3, 4) first described the cytoarchitectural organization of the spinal cord gray matter in the cat spinal cord. The gray matter was subdivided into ten layers or laminae (Fig. 1-4C). This organizational map has been applied to the spinal cord of other mammals, including humans. The histological differences seen in the gray matter correspond to functional differences (Table 1-1). Laminae I through V are found in the dorsal horn, laminae VI and VII comprise the intermediate zone gray matter, laminae VIII and IX make up most of the ventral horn, and lamina X surrounds the central canal.
Many of the neurons within the spinal cord are spinal interneurons, neurons whose synaptic connections are completely within the spinal cord. Projection neurons are neurons whose cell bodies are located in the spinal cord and that send their axons out of the spinal cord rostrally into the brainstem and cerebellum. Spinal motor neurons are neurons in the ventral spinal cord that send their axons via the ventral root directly to muscles in the periphery. In lamina IX of the spinal cord there are two types of spinal motor neurons. The larger alpha (α) motor neurons innervate the extrafusal fibers of striated skeletal muscles. The smaller and less numerous gamma (γ) motor neurons innervate intrafusal muscle fibers of the neuromuscular spindles. Information from the brain ultimately reaches motor neurons in the ventral (anterior) horn gray matter and leaves the spinal cord via the ventral roots to innervate appropriate muscles. Preganglionic sympathetic neurons supplying the entire body lie in a column of cells known as the intermediolateral cell column located in spinal segments T1 through L3. Their axons exit the spinal cord via the ventral roots (Fig. 1-5). Cells in a similar location in S2 to S4 make up the sacral parasympathetic nuclei, and their axons leave the spinal cord in the ventral roots to synapse on postganglionic parasympathetic neurons in the pelvic viscera.
Afferent information from the body is transmitted to the spinal cord via the dorsal root ganglia and dorsal roots into the dorsal (posterior) horn for processing and relay to higher brain levels. The dorsal root at each spinal cord segment consists of six to eight rootlets. The axons in each rootlet are divided into two divisions. The lateral division contains mostly unmyelinated (group C) and many finely myelinated (small group A) axons that convey sensory information related to pain and temperature. These axons enter the dorsolateral (Lissauer) tract and send ascending and descending branches that give off
collateral axons as they enter the dorsal horn of the spinal cord. The majority of these collateral axons terminate in the spinal cord segment in which they enter, but the branching can extend many segments rostral and caudal to their level of entry. These small diameter afferents terminate mainly in laminae I, II, and V of the gray matter. The medial division of the dorsal root consists of larger caliber faster conducting axons (large group A) carrying sensory information related to discriminative touch and conscious proprioception. As these axons enter the spinal cord they send collateral axons into the gray matter of the segment where they enter the spinal cord and also send branches rostral and caudal to the segment of entry. Locally these axons terminate in the deeper layers of the dorsal horn (laminae III and IV), and some branches terminate on motor neurons in the ventral horn (lamina IX) and are part of the stretch reflex.
collateral axons as they enter the dorsal horn of the spinal cord. The majority of these collateral axons terminate in the spinal cord segment in which they enter, but the branching can extend many segments rostral and caudal to their level of entry. These small diameter afferents terminate mainly in laminae I, II, and V of the gray matter. The medial division of the dorsal root consists of larger caliber faster conducting axons (large group A) carrying sensory information related to discriminative touch and conscious proprioception. As these axons enter the spinal cord they send collateral axons into the gray matter of the segment where they enter the spinal cord and also send branches rostral and caudal to the segment of entry. Locally these axons terminate in the deeper layers of the dorsal horn (laminae III and IV), and some branches terminate on motor neurons in the ventral horn (lamina IX) and are part of the stretch reflex.
Spinal Cord White Matter Tracts
The white matter is composed of longitudinally arranged myelinated and unmyelinated axons and subdivided into three regions: the dorsal (or posterior), lateral, and ventral (or anterior) funiculi. Within the funiculi long tract axons can ascend or descend the length of the spinal cord, connecting the brain and spinal cord. The funiculi also contain propriospinal axons connecting spinal cord segments that are close together or far apart (Fig. 1-4).
The dorsal funiculus contains mostly ascending axons from first-order neurons of the dorsal root ganglia (the dorsal column pathway). The afferents from segments T6 and rostral ascend more laterally in the fasciculus cuneatus, while afferents from segments caudal to T6 ascend medially in the fasciculus gracilis. The fasciculi gracilis and cuneatus are also referred to as the dorsal (posterior) columns. Between the dorsal and lateral funiculus, at the lateral edge of the dorsal horn, is the Lissauer tract (fasciculus dorsolateralis), a region of white matter that contains finely myelinated and unmyelinated primary afferent axons entering lamina II (substantia gelatinosa). The lateral and ventral funiculi contain both ascending and descending tracts. The positions of these tracts have been determined from clinical and pathological studies and from comparison with experiments conducted in animals (Fig. 1-4). Of the described pathways, the dorsal column, spinothalamic,
spinocerebellar, corticospinal, reticulospinal, rubrospinal, and vestibulospinal pathways are somatotopically arranged.
spinocerebellar, corticospinal, reticulospinal, rubrospinal, and vestibulospinal pathways are somatotopically arranged.
![]() FIGURE 1-4. The structure of the human spinal cord represented in cross section at the level of the eighth cervical segment (C8). By convention, the ventral surface of the spinal cord is oriented inferiorly. A: The left half of the spinal cord is shown stained for myelin. Heavily myelinated axons in the white matter stain darkly for myelin while the butterfly-shaped gray matter in stained less strongly. The superficial dorsal (posterior) horn and the gray matter around the central canal contain very little myelin. B: The right half of the spinal cord shown after staining for Nissl substance. The density of cell bodies in the gray matter is much higher than in the white matter. The cell bodies of large motor neurons can be seen in the ventral (anterior) horn. C: A drawing of the major anatomical features present in the spinal cord. Many of the major axon tracts are represented on the left side of the drawing. The right side shows the lamination of the spinal cord gray matter as described by Rexed (3,4) and detailed in Table 1-1. |
Table 1-1 Laminar Organization of the Spinal Cord Gray Matter | ||||||||||||||||||||||||||||||||||||||||||||
---|---|---|---|---|---|---|---|---|---|---|---|---|---|---|---|---|---|---|---|---|---|---|---|---|---|---|---|---|---|---|---|---|---|---|---|---|---|---|---|---|---|---|---|---|
|
Dorsal Funiculus
The majority of axons in the dorsal funiculus originate from dorsal root ganglia. There is also a component arising from neurons within the dorsal horn. As the tracts ascend the spinal cord axons from each spinal cord segment are added to the lateral side of the funiculus. As a result, in the upper cervical spinal cord the lowest levels of segmental innervation are located in the most medial part of the funiculus. Many of these axons convey sensory information about discriminative touch sensations and the ability to detect changes in the positions of tactile stimuli to the skin. This information is conveyed directly to nuclei in the caudal brainstem—the cuneate nucleus receives information from the body above T6 via the fasciculus cuneatus; the gracilis nucleus receives information from the body below T6 via the fasciculus gracilis. Conscious awareness of joint movement and position sense of a limb (proprioception) is conveyed from muscle spindles and, to a lesser degree, Golgi tendon organs. This information from the upper limb is carried ipsilaterally to the external or lateral cuneate nucleus in the brainstem. Similar information from the lower limb is sent to the ipsilateral nucleus dorsalis of Clarke within the thoracic spinal cord, and second order axons from these cells ascend the spinal cord ipsilaterally as the dorsal spinocerebellar tract in the lateral funiculus.
Within the dorsal funiculus there are also axons that descend the spinal cord. These axons arise from three sources: neurons in the gracile and cuneate nuclei, spinal cord gray matter, and dorsal root ganglia. The descending axons from the dorsal column nuclei form connections throughout the spinal cord, allowing the modification of sensory information sent from the spinal cord to the brain. Spinal to spinal axons can run almost the entire length of the spinal cord in both directions and could be involved in reflex coordination between the upper and lower limbs. Descending branches of primary afferents provide a means of communication between adjacent segmental levels of the body and may be part of a system that allows the regulation of signals to eventually be transmitted to the brain through long ascending tracts. Some of the long descending primary afferent axons of the dorsal funiculi are organized in distinct bundles: the fasciculus septomarginalis, adjacent to the dorsal septum, and the fasciculus interfascicularis, between the gracile and cuneate fasciculi.
Lateral Funiculus
The lateral corticospinal tract is located in the dorsal portion of the lateral funiculus and is comprised of axons from neurons in the contralateral frontal and parietal lobes of the cerebral cortex (Fig. 1-6). The axons of this tract arise from layer 5 cells of the precentral cortical area (area 4), the premotor and supplementary motor areas (area 6), and the postcentral gyrus (areas 3, 1, and 2). Approximately 90% of these axons decussate at the medullary pyramid before entering the lateral funiculus. There is also a very small component (2%) of corticospinal axons that do not cross at the medullary pyramid
and synapse on ipsilateral neurons throughout the spinal cord. The tract is known as the uncrossed lateral corticospinal tract. Corticospinal fibers from the frontal cortex synapse mainly in the intermediate gray matter and the ventral horn. Fibers from parietal cortex end in the dorsal horn. The lateral corticospinal tract is somatotopically organized so that fibers directed for the most caudal levels of the spinal cord are located most laterally in the tract. This tract controls voluntary movement of the contralateral limbs.
and synapse on ipsilateral neurons throughout the spinal cord. The tract is known as the uncrossed lateral corticospinal tract. Corticospinal fibers from the frontal cortex synapse mainly in the intermediate gray matter and the ventral horn. Fibers from parietal cortex end in the dorsal horn. The lateral corticospinal tract is somatotopically organized so that fibers directed for the most caudal levels of the spinal cord are located most laterally in the tract. This tract controls voluntary movement of the contralateral limbs.
Axons of the raphespinal tract originate from the nucleus raphe magnus in the reticular formation of the medulla and terminate in laminae I, II, and III of the spinal cord. This tract of unmyelinated axons is located in the most dorsal part of the lateral funiculus. Many of these axons contain serotonin, which may be used as a neurotransmitter in this system. The raphespinal tract acts to modify transmission from the dorsal horn of impulses initiated by noxious or painful stimuli.
The rubrospinal tract arises from the red nucleus in the rostral midbrain tegmentum. The axons from this nucleus cross in the ventral midbrain (the ventral tegmental decussation) and descend to the contralateral spinal cord. Rubrospinal tract axons are somatotopically arranged such that cervical spinal cord segments receive fibers for the dorsal part of the red nucleus, which receives input from the upper limb region of the sensorimotor cortex. Similarly, lumbosacral spinal cord segments are contacted by axons from the ventral half of the red nucleus, which receives input from the leg region of sensorimotor cortex. Rubrospinal axons synapse on interneurons in spinal cord layers V through VII. These neurons in turn project to motor neurons in the ventral horn. Many of the projections and functions of the rubrospinal tract have been defined from rodent studies. This tract may in fact not be of clinical significance since it has not been shown to project past the most rostral cervical spinal cord segments in primates and humans (5).
The major descending tract in the ventral portion of the lateral funiculus is the medullary (lateral) reticulospinal tract. It is derived mainly from the gigantocellulare reticular nucleus of the medulla. Most of the fibers are uncrossed but a small proportion cross the midline in the medulla. Whereas the corticospinal tract controls skilled voluntary movements, the reticulospinal tracts control activities that do not require constant conscious control. It is also responsible for the suppression of extensor spinal reflex activity.
Axons of neurons located in the Clarke nucleus ascend ipsilaterally forming the dorsal (posterior) spinocerebellar tract and reach the cerebellum via the inferior cerebellar peduncle. The tract is seen most caudally at L2 and increases in size until it reaches C8. Afferent fibers in segment caudal to L2 ascend in the fasciculus gracilis and synapse in the Clarke nucleus at L2. The dorsal spinocerebellar tract transmits information about muscle spindle and tendon afferents from the ipsilateral lower limb and provides the cerebellum with information about the status of individual as well as groups of muscles, thus enabling the control of movement of lower limb muscles and posture.
The ventral (anterior) spinocerebellar tract is located in the superficial ventrolateral funiculus. It originates from neurons at the base of the dorsal horn and the spinal border cells that are located along the lateral edge of the ventral horn in the lumbosacral spinal cord. Most of the axons cross the spinal cord at the level of origin and ascend contralaterally. The tract ascends as far as the midbrain and then the axons cross the midline for a second time to enter the cerebellum through the superior cerebellar peduncle. Both spinocerebellar tracts therefore transmit sensory information from one side of the body to the same side of the cerebellum.
The spinothalamic tract forms in the ventral portion of the lateral funiculus from ascending axons of neurons located in the gray matter of the opposite side of the spinal cord (Fig. 1-7). The cells of origin are in the nucleus proprius (laminae IV to VI) as well a smaller contribution from cells in laminae I, VII, VIII, and X. The axons grow across the midline in the ventral white commissure near the central canal and through the contralateral ventral horn to enter the ventrolateral and ventral funiculi. The spinothalamic tract carries information regarding tactile, thermal, and painful sensations. Its fibers are somatotopically arranged with those for the lower limb lying most dorsolaterally and those for the upper limb are more ventromedial. The main pathway in the spinothalamic tract connects second order neurons of the spinal cord gray matter with the ventral posterolateral nucleus of the thalamus (Fig. 1-8). Other axons running within the
spinothalamic tract form multisynaptic connections with several locations in the brain including midline and intralaminar thalamic nuclei, reticular formation, hypothalamus and limbic system and are involved in the activation of pain-inhibiting mechanisms (Fig. 1-8, Table 1-2).
spinothalamic tract form multisynaptic connections with several locations in the brain including midline and intralaminar thalamic nuclei, reticular formation, hypothalamus and limbic system and are involved in the activation of pain-inhibiting mechanisms (Fig. 1-8, Table 1-2).
There are two other small tracts that ascend the spinal cord in the ventral portion of the lateral funiculus. The spinotectal or spinomesencephalic tract originates from cells in laminae IV to VIII. Their axons cross the midline and then project rostrally to the periaqueductal gray matter, superior colliculus, and several nuclei in the reticular formation of the midbrain. The spinoreticular tract also originates in laminae IV to VIII and includes crossed fibers that terminate in the pontine reticular formation and uncrossed fibers that terminate in the medullary reticular formation. Many of these axons are thought to be collateral branches of spinothalamic axons. They are part of the ascending reticular activating system and may be involved in the perception of pain and of various sensations that originate in internal organs (Fig. 1-8, Table 1-2).
Table 1-2 Direct and Indirect Pathways of Pain Transmission from the Body | |||||||||||||||||||||
---|---|---|---|---|---|---|---|---|---|---|---|---|---|---|---|---|---|---|---|---|---|
|
Ventral Funiculus
The vestibulospinal tract is an uncrossed pathway originating from neurons of the lateral vestibular (Deiters) nucleus in the medulla. These axons leave the tract and enter the spinal cord at all levels and synapse on neurons in laminae VII and VIII and also terminate directly on motor neurons at the cervical and lumbar levels. It is the principal route by which the vestibular system brings about postural changes to compensate for tilts and movements of the body.
Within the medial aspect of the pontine reticular formation are two groups of neurons: the nucleus reticularis pontis caudalis (caudal pontine reticular nucleus) and nucleus reticularis pontis oralis (oral pontine reticular nucleus), which give rise to the pontine (medial) reticulospinal tract. These neurons project ipsilaterally along the entire spinal cord with their principal function being the facilitation of extensor spinal reflexes.
Another important nucleus located in the brainstem that projects to the spinal cord is the locus coeruleus (ceruleus). This nucleus is located dorsolateral to the oral pontine reticular nucleus. Neurons of the locus coeruleus contain the neurotransmitter norepinephrine (noradrenaline). The axons of these neurons branch widely and
project to virtually all regions of the CNS, including the cerebral cortex, diencephalons, brainstem, cerebellum, and spinal cord. Axons projecting to the spinal cord are diffusely scattered in the ventral and lateral funiculus.
project to virtually all regions of the CNS, including the cerebral cortex, diencephalons, brainstem, cerebellum, and spinal cord. Axons projecting to the spinal cord are diffusely scattered in the ventral and lateral funiculus.
The ipsilateral and contralateral medial vestibulospinal nucleus projects to the cervical spinal cord as part of the medial longitudinal fasciculus (MLF). Descending axons of the MLF are located in the dorsal part of the ventral funiculus and project mainly to upper cervical spinal cord segments. These axons monosynaptically inhibit motor neurons located in the upper cervical spinal cord. It is responsible for stabilizing the head position during movement. In the brainstem, many secondary vestibular fibers project directly through the MLF to the motor neurons of the oculomotor, trochlear, and abducens nuclei, forming the basis of the vestibulo-ocular reflex. A small group of descending axons entering the spinal cord and, running in the superficial ventromedial portion of the ventral funiculus, come from neurons in the superior colliculus and form the tectospinal tract. These axons terminate in laminae VI, VII, and VIII in rostral segments of the cervical spinal cord and are involved with directing head movements in response to visual and auditory stimuli.
Another component of corticospinal fibers, the ventral (anterior) corticospinal tract, is located in the most medial portion of the ventral funiculus and is compose of 8% of corticospinal axons that did not cross at the medullary pyramid. These axons descend the spinal cord and eventually cross the midline of the spinal cord at different segmental levels to synapse on cells in the contralateral ventral horn.
Propriospinal Tract
In all three funiculi, the white matter immediately adjacent to gray matter contains the majority of propriospinal axons, both ascending and descending within the spinal cord. Shorter axons are found closer to the gray matter than longer fibers. Some of these axons can cross the midline of the spinal cord, while others remain ipsilateral. They connect different segments of the spinal cord and mediate intrinsic reflex mechanisms of the spinal cord including the coordination of upper and lower limb movements.
Histology and Pathophysiology of Acute Spinal Cord Injury
Experimental Models of Spinal Cord Injury
Cases of SCI that involve gunshot or stab injuries (sharp trauma) may result in complete severing of the spinal cord, but in most cases patients present with an anatomically incomplete lesion of the spinal cord. Compression or contusion injury (blunt trauma) is more frequent, occurring as a result of fracture or subluxation of the vertebrae. These types of injury can cause contusion and laceration of the spinal cord. Animal models have been developed to better study the events following SCI. These have been very important in understanding the cause, effect, and sequence of events, especially in the acute phase of injury (6, 7 and 8). Many different animal species have been used to model SCI, with the majority of the studies carried out in rodents. A wide range of surgical approaches have also been developed to investigate SCI, most involve exposing the spinal cord with a laminectomy and then either surgically transecting specific tracts or regions of the spinal cord or creating a contusion injury using one of several types of weight drop devices (Fig. 1-9) (6). Other models have created SCI within the closed vertebral column by inserting and inflating a balloon (9, 10 and 11) or the intravascular injection of a photosensitive dye, which is then activated in the vessels of the spinal cord, causing local vascular damage and subsequent injury (12 and 13). Care must be taken in interpreting any animal model of SCI; not only in using the results to better understand human injury but also in recognizing that the animal species and strain chosen for an experiment can influence the outcomes (14).
Hemorrhage and Edema
SCI has been described as developing in three phases: acute, secondary, and chronic (15, 16, 17 and 18). The direct mechanical trauma to the spinal cord is quickly followed by local ischemia and hemorrhage, resulting in edema. These events cause neural cells in the immediate vicinity to die, resulting in the loss of local synaptic contact and blockage of signal transmission through the injury site. Multiple hemorrhage points appear within minutes of the insult and increase over the next several hours (15, 19, 20). The physical breakdown of the microvasculature leads to a loss of the blood-brain barrier and leakage of fluid into the spinal cord. Edema is seen for the first few days following injury. The increased fluid resulting from edema can further compress the spinal cord, causing more edema and thus additional damage. Several treatments to reduce edema have been tried experimentally, leading to some improvement (21, 22, 23 and 24).
Spinal Shock
The term spinal shock was introduced to describe a patient’s condition following acute SCI; muscle paralysis, flaccid muscle tone, and loss of tendon reflexes below the level of the lesion (25). Spinal shock worsens in degree and duration with increasing severity of the injury (26), and, if untreated, it can last for up to 6 weeks. During this
time the brain and rostral spinal cord cannot communicate with the spinal cord below the level of damage. This will make the loss of function below the injury appear complete, and it is only once the swelling associated with the injury subsides that the true extent of the damage can be determined. During the months after SCI, spasticity develops with exaggerated tendon reflexes, increased muscle tone, and muscle spasms. A hypothesis has been developed to explain this dramatic change in symptoms. A change from hypoexcitability of α-motor neurons during spinal shock to hyperexcitability during spasticity is presumed (27). According to this theory, the α-motor neuron hypoexcitability in spinal shock is due to an acute loss of supraspinal excitatory input, resulting in a hyperpolarized α-motor neuron (28). Hyperpolarization of spinal motor neurons has been observed in spinal cords of transected (spinalized) cats (29). H reflexes, which are elicited with electrical stimulation of the nerve as opposed to the tendon reflex, which is secondary to mechanical stretch, have been described in humans early after SCI (25, 30); this finding argues against the theory of α-motor neuron depression as the single reason for the loss of tendon reflexes during spinal shock. This led to the idea of coincident γ-motor neuron depression since even though a physical tap does not elicit a tendon tap reflex, electrical stimulation of group I afferent nerve fibers leads to an H-reflex response (25). The interruption of descending noradrenergic (locus coeruleus) and serotonergic (raphe nucleus) pathways from the brainstem could also be involved (31, 32 and 33).
time the brain and rostral spinal cord cannot communicate with the spinal cord below the level of damage. This will make the loss of function below the injury appear complete, and it is only once the swelling associated with the injury subsides that the true extent of the damage can be determined. During the months after SCI, spasticity develops with exaggerated tendon reflexes, increased muscle tone, and muscle spasms. A hypothesis has been developed to explain this dramatic change in symptoms. A change from hypoexcitability of α-motor neurons during spinal shock to hyperexcitability during spasticity is presumed (27). According to this theory, the α-motor neuron hypoexcitability in spinal shock is due to an acute loss of supraspinal excitatory input, resulting in a hyperpolarized α-motor neuron (28). Hyperpolarization of spinal motor neurons has been observed in spinal cords of transected (spinalized) cats (29). H reflexes, which are elicited with electrical stimulation of the nerve as opposed to the tendon reflex, which is secondary to mechanical stretch, have been described in humans early after SCI (25, 30); this finding argues against the theory of α-motor neuron depression as the single reason for the loss of tendon reflexes during spinal shock. This led to the idea of coincident γ-motor neuron depression since even though a physical tap does not elicit a tendon tap reflex, electrical stimulation of group I afferent nerve fibers leads to an H-reflex response (25). The interruption of descending noradrenergic (locus coeruleus) and serotonergic (raphe nucleus) pathways from the brainstem could also be involved (31, 32 and 33).
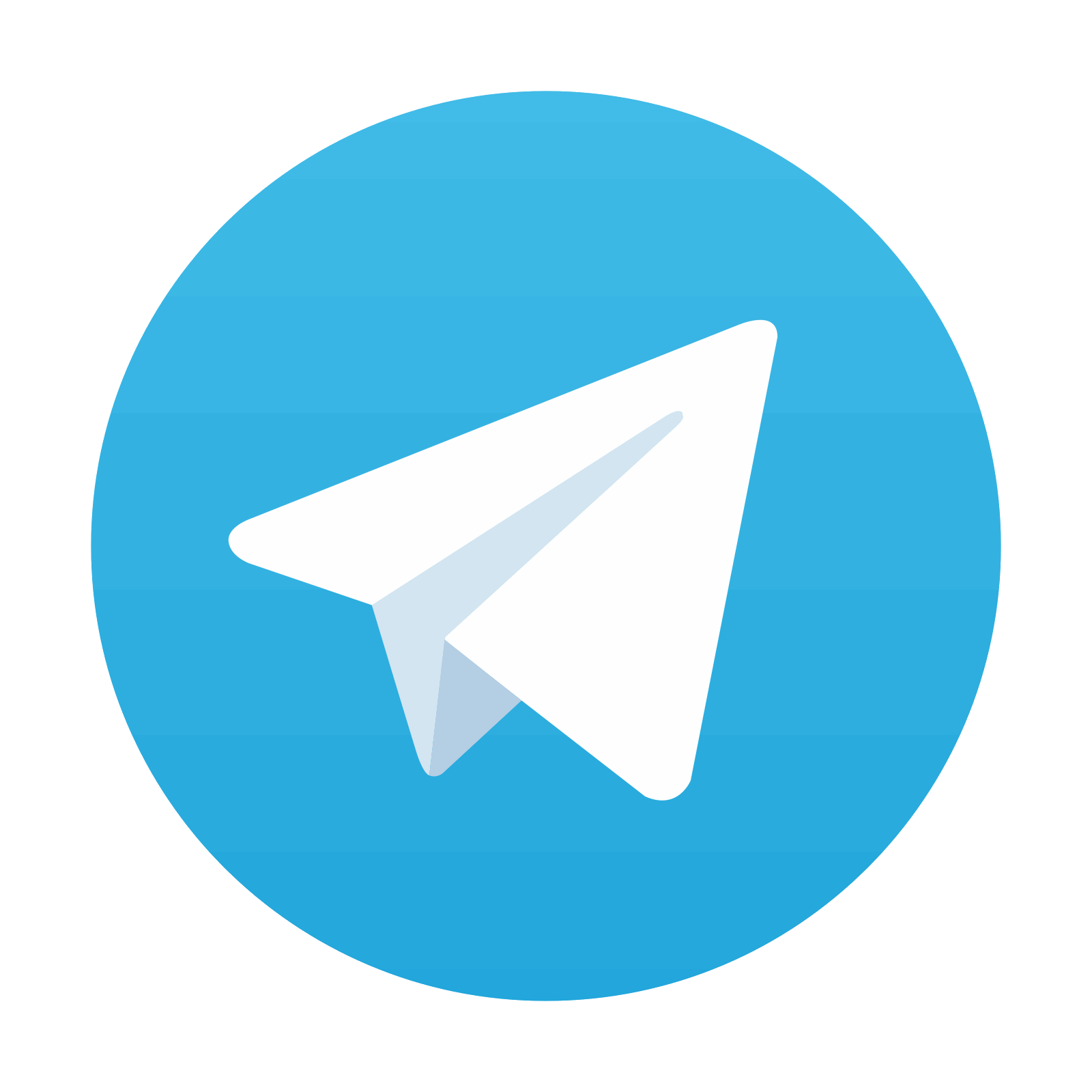
Stay updated, free articles. Join our Telegram channel

Full access? Get Clinical Tree
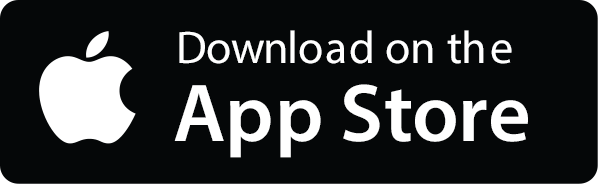
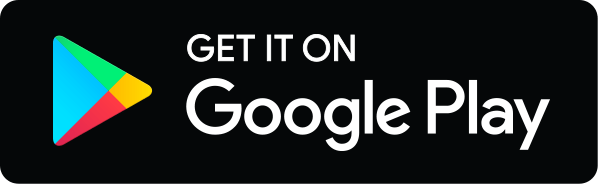