Fig. 21.1
Ossification centers of the atlas and axis (Reprinted from Copley and Dormans [15]. With permission from Wolters Kluwer Health)
Distinctive anatomic features in the immature C-spine account for the pattern of injuries seen in this population. The horizontal orientation of the vertebral facet joints in the pediatric cervical spine and the relative ligamentous laxity results in a relatively high incidence of upper cervical subluxation and cervical injuries in this population. Before 8 years of age, the articular facets have an orientation of ~30° and progressively become more vertical. By adolescence, the orientation is similar to that of an adult at 55–70° [1]. Furthermore, the relatively high head-to-body ratio of the pediatric patient localizes the fulcrum of spinal flexion at C2–C3 as opposed to C5 and C6 in the adult [17]. Other anatomic features contributing to the pattern on injuries seen in this population include delayed ossification of the uncinate process (a stabilizer in the adult spine), anterior wedge of the immature vertebral body, and underdevelopment of cervical para-spinal muscles. These factors contribute to the relatively high incidence of upper cervical instability/injuries in the pediatric patient [1, 18, 19].
21.2.3 Clinical Evaluation
Cervical spine injury in the pediatric patient often results from considerable trauma such as motor vehicular accidents, fall from height, and penetrating injuries. Athletic injuries and child abuse are also potential causes of trauma in the pediatric spine. Patients may be unconscious at presentation. When conscious, they report neck pain or spasm. Signs of trauma to the head and face, bruising caused by seat or shoulder belts may also be present. Often these findings are absent in the young child, and instead, the child may report occipital headaches. Additionally, increased suspicion is crucial in children with syndromes that have a genetic predisposition for cervical spine injuries like Down syndrome, Klippel-Feil syndrome, and Morquio syndrome.
In addition to strict adherence to ATLS protocol, a comprehensive neurological examination should be performed on all patients with suspected spinal injuries, the patient should also evaluated for other associated injuries [21].
The child presenting with a suspected cervical spine injury should be immobilized in a spine board. The indications proposed by Lee at al. [22] for immobilization of the pediatric cervical spine are presented in Table 21.1.
Table 21.1
Indications for immobilization of the pediatric cervical spine
Abnormal neurological examination findings (complete testing of motor, sensory, and reflex functions of all extremities is required) |
Unreliable examination due to inconsolable or unconscious children, or substance abuse |
Neck pain or focal neck tenderness |
Mechanism of injury potentially associated with CSI (high-speed motor vehicle collisions, falls greater than body height, bicycle or diving accidents, forced hyperextension injuries, acceleration–deceleration injuries involving the head) |
History of transient neurological symptoms suggestive of SCIWORA (weakness, paresthesias, or lightening/burning sensation down the spine/extremity or related to neck movement) |
Physical signs of neck trauma (ecchymosis, abrasion, deformity, swelling, or tenderness) or significant trauma to the head or face |
Because of the relatively large head of children <8 years of age, there is a tendency for flexion of the head, when these patients are immobilized in a traditional spine board. This can be prevented by elevating the torso relative to the head with a thin mattress or using a board with a recess [23]. A halo ring and vest is also a viable method of immobilizing the pediatric cervical spine. The construct requires the use of 8–12 pins applied with low insertional torques (1–5 in. lbs). A CT scan of the head obtained prior to application of the halo can help identify cranial sutures and thin areas of the skull. These areas should be avoided when inserting halo pins. Advantages of a halo vest immobilization include relative ease of application, earlier mobilization of the patient, access to wounds of the neck and scalp, and freedom of mandibular motion. Pin site infection is the most common complication associated with halo vest immobilization [24, 25].
21.2.4 Radiological Evaluation
The standard trauma series, including an AP, lateral, and odontoid views of the cervical spine, including the T1 level, can be obtained when a C-spine injury is suspected. In the lucid and older patient without neurological deficits, flexion and extension views of the C-spine should also be obtained if instability is suspected. Indices of instability include segmental kyphosis, anterior soft tissue swelling, and equivocal subluxation in the standard radiographic views [1, 26]. In small infants, odontoid views and lateral views may be difficult to interpret because of the overlapping skull. In these instances, a lateral view of the skull may be utilized to evaluate the upper cervical spine [1].
Radiographic parameters used for the evaluation of upper cervical stability include the atlanto-dens interval (ADI), Powers ratio, and the basion-axis distance. The ADI describes the distance between the anterior surface of the dens and the posterior surface of the anterior arc of the atlas and should be <5 mm. The Powers ratio defines the ratio between the distance of the basion and the posterior arc of the atlas, and the distance between the opisthion and the anterior arc of the atlas. This ratio averages 0.77 and a value exceeding 1 or <0.55 is indicative of atlanto-occipital dislocation. The basion-axis distance is the distance between the basion and a vertical line extended cephalad from the posterior border of the dens. This line should be 12 mm or less in children 13 years of age or less (Fig. 21.2) [19, 21].
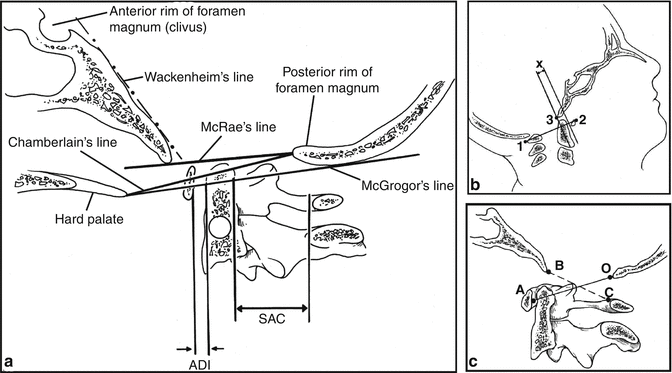
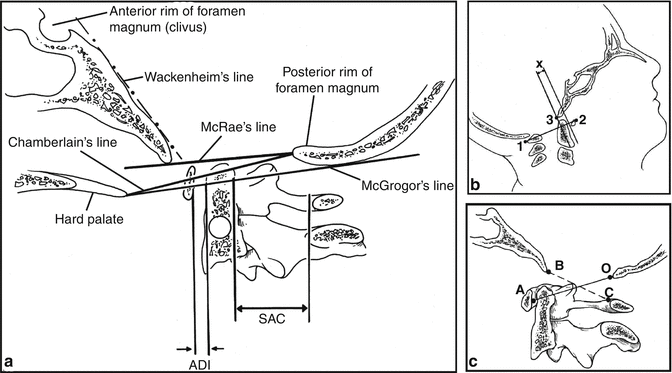
Fig. 21.2
Cervical spine landmarks and measurement parameters. (a) The lines commonly used to determine basilar impression and the measurements for determining atlantoaxial instability. ADI atlanto-odontoid interval, SAC space available for cord. (b) Method of measuring atlanto-occipital instability according to Wiesel and Rothman [27]. The atlantal line joins points 1 and 2. A line perpendicular to the atlantal line is made at the posterior margin of the anterior arch of the atlas. The distance (x) from the basion (3) to the perpendicular line should not vary by 1 mm or more in flexion and extension. (c) The ratio of powers is determined by drawing a line from the basion (B) to the posterior arch of the atlas (C) and a second line from the opisthion (O) to the anterior arch of the atlas (A). The length of line BC is divided by the length of line OA. A ratio of 1.0 or greater is diagnostic of anterior occipitoatlantal dislocation (Reprinted from Copley and Dormans [15]. With permission from Wolters Kluwer Health)
Normal anatomical variants in the developing spine should be recognized and differentiated from pathological findings. The most common anatomical variation in the pediatric spine is the physiological displacement between C2 and C3, and less often C3 and C4; pseudosubluxation of C2 on C3 was observed in 22 % of pediatric polytrauma patients by Shaw et al. [28] and in 46 % on lateral dynamic views in patients <8 years of age by Cattell and Filtzer [29]. In order to differentiate this pseudosubluxation from an injury, one must evaluate the spinolaminar line (i.e., Swischuck’s line) (Fig. 21.3). The spinolaminar line is drawn along the posterior arch of C1 to C3 and normally passes within 1 mm of the anterior cortex of the posterior arch of C2. That said, a displacement of >1.5 mm should raise concerns and a displacement of >4 mm is always an abnormal finding [29].
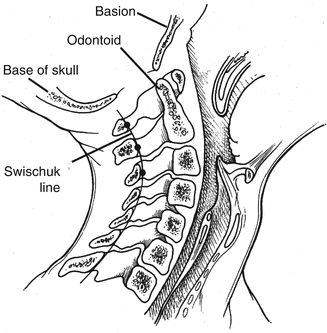
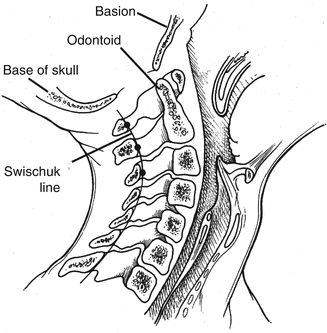
Fig. 21.3
Spinolaminar line (Swischuck line) used to differentiate pseudosubluxation of C2 on C3 from true cervical injury (Reprinted from Copley and Dormans [15]. With permission from Wolters Kluwer Health)
Absence of cervical lordosis, a pathological finding in the adult patient, may be normal variant in some pediatric patients younger than 16. In these patients, an intraspinous distance of 1.5 times the distance of the adjacent intraspinous distances or less confirms stability [29, 30]; additionally, normal lordosis is restored with the neck in extension.
In the subject of normal CT measurements and parameters specifically of the pediatric cervical spine, recent literature suggests the need to define age- and sex-specific values. Vachhrajani et al [20] found that there are age-independent (LMI = lateral mass interval and ADI = atlantodental interval) but also age-dependent (BDI = basion-dental interval; CCI = craniocervical interval, and PADI = posterior ADI) normal CT measurements of the upper cervical spine in children [21]. Further research is needed to validate these preliminary values in a larger and geographically diverse patient cohort so as to better direct patient care.
Differentiation of true fractures from unfused physeal line is essential in the evaluation of the pediatric spine. Secondary ossification centers in the spinous processes and unfused ring apophyses of vertebral bodies may mimic fractures on radiographs. Normal physeal plates are smooth, regular areas of radiolucency with underlying subchondral sclerotic lines and occurring at predictable locations. In contrast, acute fractures are irregular, without sclerosis, and occur at any location within the C-spine [21, 30].
Finally, although advanced imaging (CT and MRI) can be used to identify subtle injuries, undetected from the physical examination and the plain radiographs, CT should not be used to clear the cervical spine of a pediatric trauma patient with a potentially existing instability secondary to soft tissue injury [31]. Additionally, since flexion and extension radiographs are inappropriate to assess instability in intubated, obtunded, or uncooperative children, MRI can be very helpful in identifying soft tissue injury. Flynn et al. [32] reported that MRI was able to demonstrate cervical injuries not seen in radiographs in 15 of 64 children (24 %). Finally, CT angiograms can be very helpful in delineating vascular compromise (Fig. 21.4).
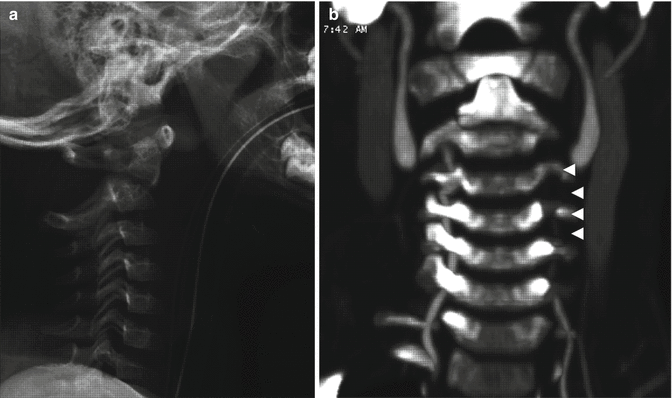
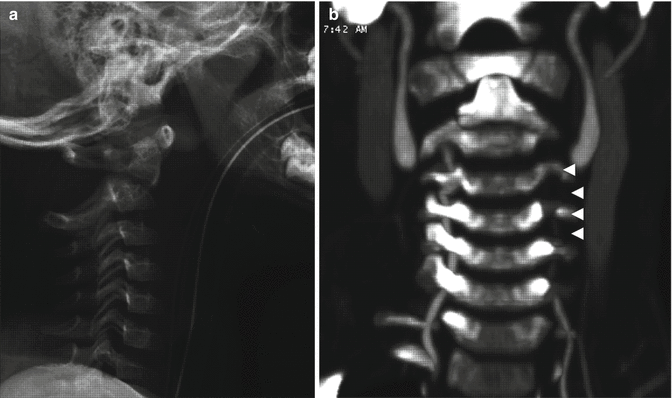
Fig. 21.4
Lateral radiograph of the cervical spine (a) of a 4-year-old male after being struck by a car demonstrating an odontoid fracture, with posterior dislocation of the C2 vertebral body with respect to the dens and increased widening of the posterior elements of C1 and C2. CT angiogram of the neck (b) demonstrating a dens fracture and accompanied compromise of the left vertebral artery with long-segment luminal irregularity (arrows)
21.2.5 Specific Injury Patterns
21.2.5.1 Atlanto-occipital Dislocations
A historically fatal injury, current management protocols have decreased the mortality associated with these injuries. These protocols include improved resuscitation at the scene of injury, early immobilization, high index of suspicion, and improved diagnostic modalities. These injuries often result from a rapid deceleration during an MVC or MVC-pedestrian collision. The head is hyperflexed relative to the torso resulting in dislocation of the atlanto-occipital joint. The lack of inherent bony stability of the atlanto-occipital joint, and ligamentous laxity of the pediatric cervical spine, predisposes the young child to this injury. Three types of atlanto-occipital dislocation (AOD) have been described. Type 1 involves anterior displacement of the occiput relative to the atlas; type 2 describes longitudinal displacement of the occiput from the atlas; and type 3 describes a posterior displacement [19].
The paired semilunar occipital condyles located at the inferior surface of the cranium articulate with the concave lateral masses of C1. At birth, the surfaces of the lateral masses are relatively flat and progressively become more concave with age. This absence of bony articular congruence contributes to the increased risk of atlanto-occipital instability in the young pediatric patient, especially under the age of 6 years [33].
Numerous ligaments provide atlanto-occipital stability. The tectorial membrane, a cranial extension of the posterior longitudinal ligament, attaches C1 to the anterior surface of the foramen magnum. Deep to the tectorial ligament are the paired alar and apical ligaments. These ligaments originate from the dens, with the former attaching to the medial aspects of the occipital condyles and the latter attaching to the foramen magnum. The tectorial, alar, and apical ligaments constitute the major stabilizers of the atlanto-occipital joint. The tectorial membrane limits extension, while odontoid impaction on the basion limits flexion. The alar ligaments limit lateral bending. Both the alar ligaments and tectorial membranes limit distraction [33].
The patient with AOD is often polytraumatized with numerous associated injuries including head injuries. Hemodynamic instability, often secondary to neurogenic shock, is a common finding. On presentation, the patients may be intubated and on pressors. The earlier clinical state often conceals the presence of an AOD and prevents a comprehensive neurological examination. High clinical suspicion is therefore needed to diagnose AODs and optimize management. Patients often present with quadriplegia or quadraparesis. In addition, cranial nerve palsies, especially the 6th, 9th–12th, are often present. The lower CN palsies may be secondary to stretch injury, while the sixth palsy may result from head injury. The vertebral arteries may also be injured [34–36].
Plain radiographs are the mainstay in the evaluation of patients with AOD. Power ratio, the Wackenheim line, and the occipitocondylar distance are radiographic parameters used in the evaluation of atlanto-occipital instability. On the lateral view, the Wackenheim line is drawn along the clivus and tangentially intersects the tip of the odontoid. Anterior or posterior displacement of this line relative to the odontoid signifies a corresponding displacement of the occiput on the axial spine. The occipital condylar distance defines the distance between the occipital condylar facet and the C1 facet. A distance greater than 5 mm signifies disruption of the atlanto-occipital joint (see Fig. 21.2) [19, 21, 30]. Finally, the power ratio is defined as the ration of the distance from the basion to the midcervical portion of the posterior laminar line of the atlas to the distance from the opisthion to the midvertical portion of the posterior surface of the anterior ring of the atlas. A ratio >1.0 is suggestive of anterior subluxation of the occiput on the atlas. CT scan is a sensitive method of evaluating atlanto-occipital instability. Fine-cut CT with coronal and sagittal reconstruction clearly demonstrates malalignment of the atlanto-occipital joints. MRI has also been utilized to evaluate atlanto-occipital instability. It provides excellent visualizations of the ligaments and soft tissue structures above the atlanto-occipital joint. Visualization of a complete defect of the tectorial membrane on MRI is diagnostic of AOD. Furthermore, injuries to the spinal cord can be visualized on MRI [33].
As with all polytraumatized patients, ventilation should be optimized and hemodynamic stability maintained. The C-spine should be immobilized in a neutral position; halo immobilization with or without traction or Minerva casting are the two prevalent options. Use of cervical traction remains controversial with some advocating its utility in type l and type 3 injuries. That said, cervical traction has no role in the management of type 2 injuries because alignment is adequate [33, 35–37].
Definitive management of AOD is controversial with many authors advocating early posterior fusion since many of these injuries ultimately become unstable due to the associated soft tissue injury. Techniques described in the literature include contoured loop fixation, atlantoaxial transarticular screw placement, and wire fixation with bone grafting. Two techniques using wire fixation and autologous iliac crest graft or rib grafts have been described. Both the procedures require the use of cables or wires affixed to two burr holes drilled on the occiput. In the former, a single-shaped iliac graft is attached to a trough prepared at the base of the occiput and to the spinous process of the axis. In the latter procedure, paired autologous rib grafts with a natural curve resembling the spinal anatomy are harvested, and fixed to the occiput and axis with sublaminar wires (Fig. 21.5a–d) [16, 34, 35]. Factors favoring a decision to proceed with immediate posterior fusion include 3 years of age or later, and a complete AOD with neurological deficits. Some have, however, recommended initial nonoperative management with an orthosis as it is believed that the pediatric spine has a higher repair capacity. Healing can thus be obtained without instrumentation. Because of the technical difficulties involved in instrumentation of the very immature spine, and the high healing capacity of very young children, nonoperative management is often a viable option in this population. Nonoperative management may also be indicated in patients with incomplete injuries [16, 33–35, 38].
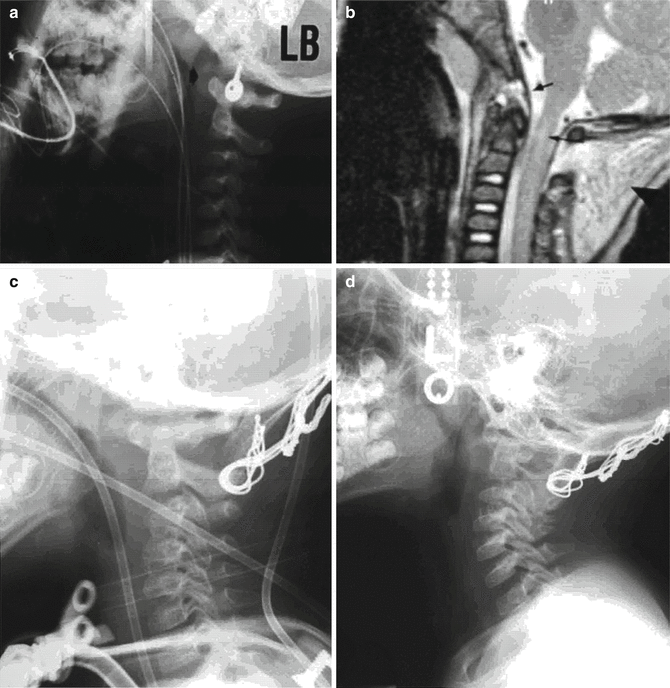
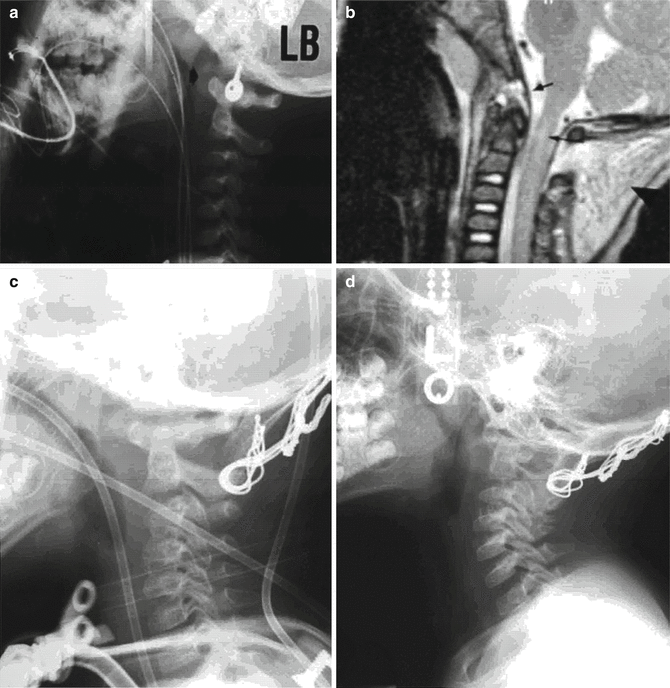
Fig. 21.5
A 1-year-old male pedestrian stuck presenting with the AOD. (a) Lateral C-spine radiographs showing an AOD and atlantoaxial disruption. (b) T2 MRI demonstrating spinal cord contusion. (c, d) Postoperative (12 and 55 weeks after posterior fusion) radiographs showing normal alignment and graft consolidation (Reprinted from Hosalkar et al. [35]. With permission from Journal of Bone and Joint Surgery)
21.2.5.2 Fractures of the Atlas
Fractures of the atlas, classically the Jefferson fracture, are uncommon injuries in the pediatric population. It is usually secondary to an axial compression load resulting from a fall onto the top of the head or hyperextension after a motor vehicular crash. Force is transmitted from the occiput to the lateral masses, resulting in a fracture at the weakest point of the atlas. This is usually the anterior or posterior arch. Diastasis of the lateral masses can also result in avulsion of the transverse ligament, leading to atlantoaxial instability. Current studies show a 6.9-mm displacement of the lateral masses and suggest a transverse ligament rupture, which result in instability [15, 39, 40].
Pediatric patients with a fracture at the atlas usually present with neck pain, cervical muscle spasm, torticollis, and reduction in range of motion. Neurological deficits are uncommon with C1 fractures. Though the earlier-mentioned signs and symptoms are nonspecific, they should raise the index of suspicion and prompt assessment for the presence of this fracture pattern especially since they are commonly missed due to inadequate imaging of the occipitocervical junction [41].
Isolated fractures of C1 is often absent on plain radiographs. Findings suggestive of these fractures include a prevertebral hematoma on the lateral cervical spine views, or separation of the lateral masses on an odontoid view. A CT scan of the C-spine and MRI is needed for accurate diagnosis of these fractures (Fig. 21.6a, b) [39, 40, 42].
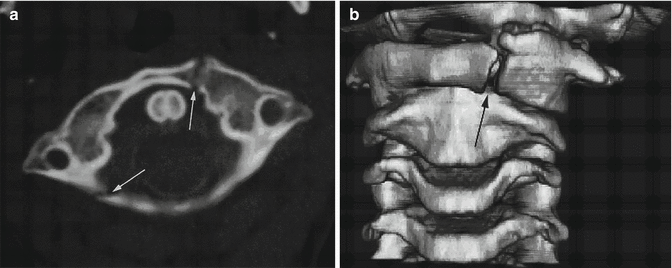
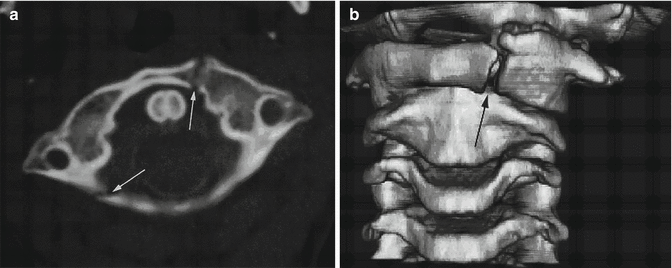
Fig. 21.6
Axial CT scan through C1 (a) and three-dimensional reformatted CT scan of the upper cervical spine (b) obtained in a 6-year-old boy showing anterior and posterior ring fractures of C1 (arrows) (Reprinted from Lustrin et al. [21]. With permission from Radiological Society of North America)
There are few reports of isolated pediatric C1 ring fractures in the literature and conservative management of these fractures with external immobilization seems to be the consensus recommended treatment. Successful use of soft cervical color, halo vest, and rigid cervical collar for varied duration has been documented. Recovery of full function and return to activities are expected with conservative management [41–45].
21.2.5.3 Odontoid Fractures
Odontoid fractures are common in the pediatric population. Like most cervical spine injuries in this population, the cause of injury often involves an MVC via rapid deceleration with flexion, or a fall from height. Odontoid fractures usually occur at the base of the dens at the synchondrosis. The weak synchondrosis and the relatively large head size of the young pediatric patient predispose this location to injury.
The clinical presentation of patients with odontoid fractures can range from neck pain to significant spinal cord injuries (SCI). Fasset et al. [46] demonstrated 33 % incidence of neurological deficits in patients presenting with odontoid fractures. When present, spinal cord injuries (SCI) tend to occur at the cervicothoracic junction. It has been proposed that this may be secondary to a traction injury of the cord resulting from hyperflexion [47]. In the Fasset et al. [46] series, 53 % of SCIs occurred at the cervicothoracic level.
Radiographic evaluation of odontoid fractures begins with a plain radiograph of the C-spine. On the lateral view, anterior angulation or displacement of the dens is often apparent. A CT scan with a sagittal and 3D reconstruction may be needed to further delineate the fracture site and demonstrate diastasis of the synchondroses. In equivocal cases, MRI imaging can be useful. Soft tissue changes at the C1 and C2 levels combined with a high signal at the synchondroses in the appropriate clinical setting suggest a fracture [21, 46, 48].
Odontoid fractures can be managed nonoperatively. Closed reduction can be obtained with extension or hyperextension of the C-spine (Fig. 21.7). Fifty percent apposition of the fracture segment is usually sufficient for healing. The C-spine is subsequently immobilized in a halo vest or pinless halo for 2–3 months. Frequent radiographic follow-up is required to insure stability is maintained. Fasset et al. [46] showed a fusion rate of 93 % with conservative management.
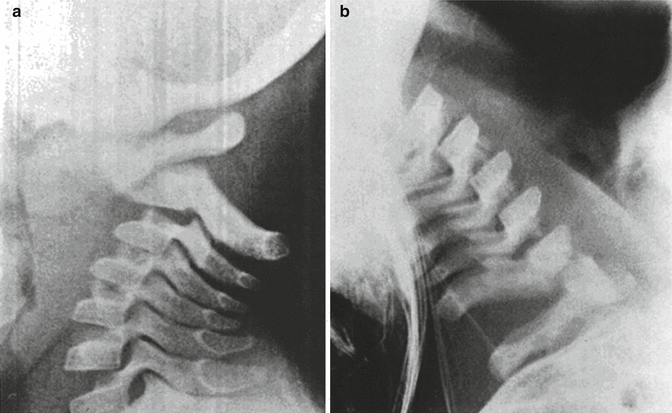
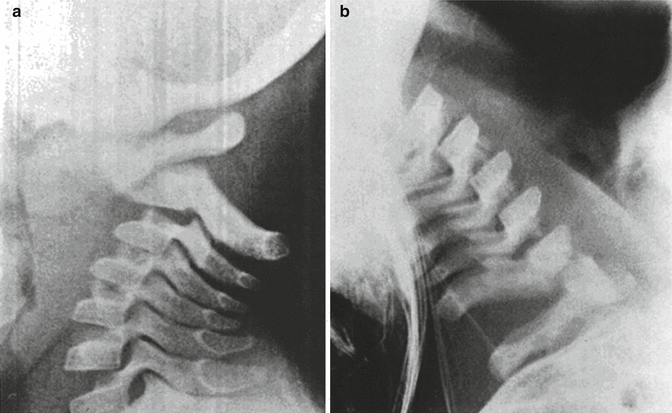
Fig. 21.7
Lateral C-spine radiographs of a young child with a severely displaced odontoid fracture after a fall. The second image shows a reduced fracture with hyperextension (Reprinted from Sherk et al. [49]. With permission from American Journal of Bone and Joint Surgery)
Operative management is indicated in fractures where reduction is not obtained with external immobilization, or with nonunion (no evidence of healing after 3–6 months). Viable surgical options include posterior C1 and C2 fusion with bone grafting and wiring. Motion-sparing procedures have not been proven to be efficacious in the pediatric population [46]
21.2.5.4 Hangman’s Fracture
Traumatic anterior spondylolisthesis of the axis due to bilateral fracture of the pars interarticularis is extremely rare in the pediatric population with only a few cases reported. It occurs most commonly in children 2 years of age and or less. Various factors account for this pattern of injury in the developing spine. As indicated earlier, the relatively large head-to-body ratio of the young child localizes fulcrum of flexion over the upper cervical spine. This is further compounded by the relative ligamentous laxity and weak neck muscles of the young child. That said, injury results from cervical hyperextension [19, 50].
Radiological diagnosis of pediatric Hangman’s fracture is complicated by unique features of the pediatric spine, as discussed earlier. Hangman’s fractures involve fractures of the pars interarticularis and anterolisthesis of C2 on C3. Evaluation of the pediatric Hangman’s fracture begins with standard plain radiographic views, with CT and MRI reserved for more detailed evaluation. On radiographs, a radiolucent line can be observed anterior to the pedicles of the axis. Pathological anterior displacement of C2 is likely if the tip of the spinous process of C2 is greater than 2 mm anterior to a line (Swischuk’s line) connecting the spinous processes of C1–C3 (see Fig. 21.2) [16, 19].
The Levine classification system for traumatic spondylolisthesis can be extrapolated to the pediatric population. Type 1 injury describes 3 mm or less translation between C2 and C3 without angulation. In type 2 injuries, there is greater than 3 mm of translation between C2 and C3 and greater than 10° angulation. Type 3 fractures include the characteristics of type 2 fractures as well as bilateral facet dislocation and have greater angular components [51].
Management of nondisplaced Hangman’s fracture is usually conservative with immobilization in a halo ring and vest or pinless halo. Levine type 3 fractures are managed surgically with open reduction and posterior fusion [19].
21.2.5.5 Atlanto-axial Rotatory Subluxation
Injuries of the atlantoaxial joint include ligamentous disruptions resulting in instability and odontoid fractures. The atlantoaxial joint is a relatively mobile joint with 50 % of cervical rotation occurring at this joint. Furthermore, its diameter, one-third occupied by the dens, one-third by the spinal cord, and one-third by subarachnoid space, allows for significant cervical motion before cord injury [21, 52].
Atlantoaxial rotatory subluxation (AARS) is a rotational deformity of C1 on C2 most commonly secondary to infection or trauma leading to lateral neck flexion and contralateral rotation. Upper respiratory infections, retro-pharyngeal abscesses are common causes of atlantoaxial instability. However, it also results from trauma in ~20–45 % of the patients [1, 19].
Children with atlantoaxial subluxation present with torticollis, with the chin rotated to one side and head laterally deviated to the contralateral side. In addition, there is spasm of the sternocleidomastoid muscle opposite to the side of chin rotation. They will typically report headaches and neck pain. In a fixed subluxation, rotation of the atlantoaxial joint is prevented or limited by impingement of the C1 facet joint on C2. An attempt at manual reduction of the deformity is usually painful. Neurological deficits are rare [1, 36, 52].
Radiographic assessment of patients presenting with suspected atlantoaxial dislocation includes standard cervical spine series complemented with a dynamic rotation CT scan. MRI is useful to rule out other diagnosis and assess further the surrounding soft tissues. Findings indicative of subluxation on lateral radiographs include absence of a defined craniocervical junction and lack of orientation of the anterior arch in a true lateral plane. On the AP plane, the anteriorly subluxated lateral mass may appear wider and more proximal to the midline, while the opposite mass appears farther and smaller. Significant displacement is however unlikely in the presence of a normal ADI. Plain radiography is often difficult to interpret because of the tilted position of the head (Fig. 21.8a–d) [19, 21].
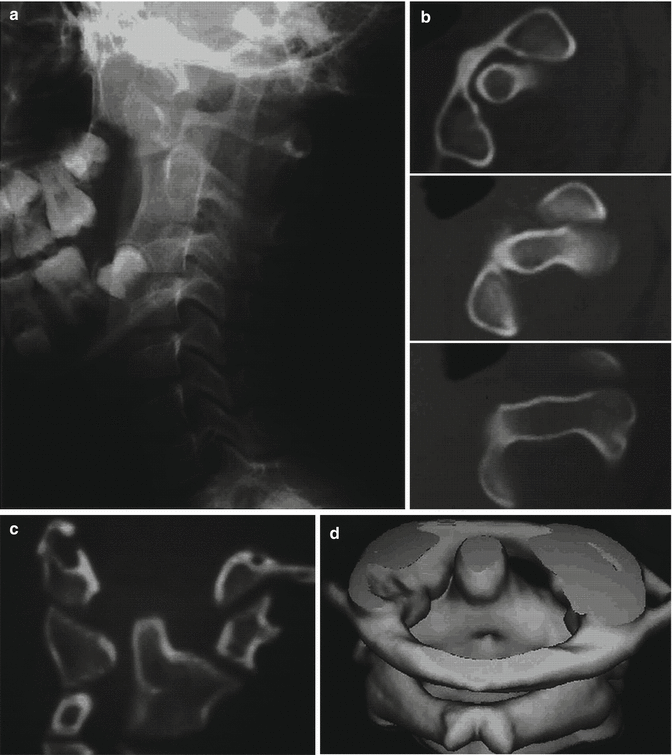
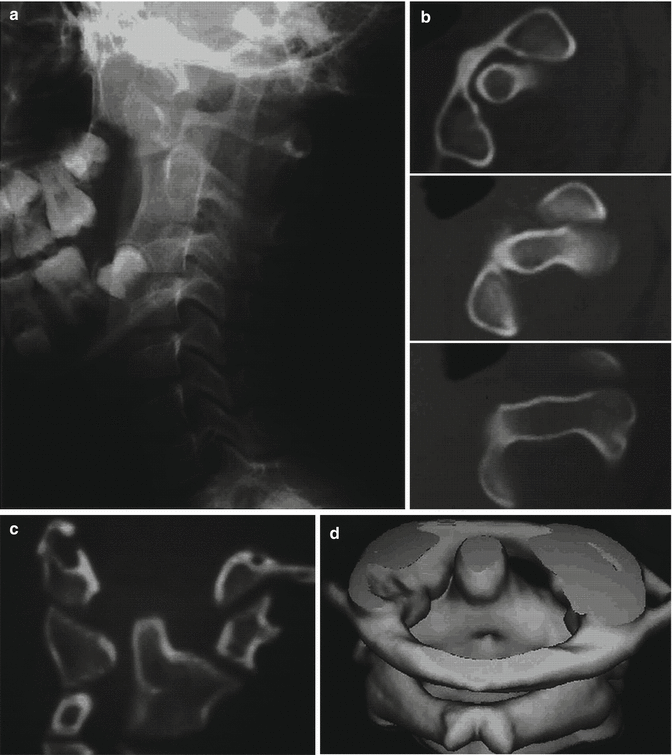
Fig. 21.8
Lateral radiograph of the cervical spine (a), axial CT scans (b), coronal CT scan (c), and 3D reformatted CT scan (d) through C1 and C2 in a 12-year-old girl with atlantoaxial rotatory subluxation (Reprinted from Lustrin et al. [21]. With permission from Radiological Society of North America)
Fielding and Hawkins [53] classified atlantoaxial rotatory subluxation (AARS) into four categories. Type 1, the most common, shows no displacement of C1 and a normal ADI. It results from unilateral facet subluxation without disruption of the transverse ligament. Type 2 demonstrates a greater than 3–5 mm anterior displacement with compromise of the transverse ligament. Type 3 describes bilateral anterior facet dislocation associated with complete rupture of the transverse ligament and leads to significantly reduced space for the spinal cord. It is manifested by a >5-mm ADI. Type 4 demonstrates posterior displacement of C1 and is rare.
Atlantoaxial rotatory fixation (AARF) can be demonstrated with a dynamic rotation CT. A fixated C1 and C2 unit rotates as one on a CT obtained at rest, and with attempted neck rotation. It results from an untreated AARS [21].
Management of atlantoaxial rotatory subluxation may involve conservative management or in rare instances surgical stabilization. Often, mild subluxations may reduce spontaneously and patients do not present for formal intervention. Patients presenting with symptoms of <1 week duration can be treated with a soft cervical collar, NSAIDs, and muscle relaxants. If symptoms have persisted for >1 week, patients may be treated with head halter traction, supplemented with muscle relaxants and analgesics. Conservative therapy is unlikely to be successful in patients presenting with symptoms persisting for >1 month. In this setting, reduction can be attempted with a halo traction or manual manipulation under anesthesia. If successful, the patient can be immobilized in a halo vest for 6 weeks. If this modality is unsuccessful, surgery may be indicated. Surgery is also indicated in the presence of persistent instability, neurological deficits and may include bilateral facet reduction with fusion. The atlantoaxial joint may also be fused in a subluxated position [19, 54].
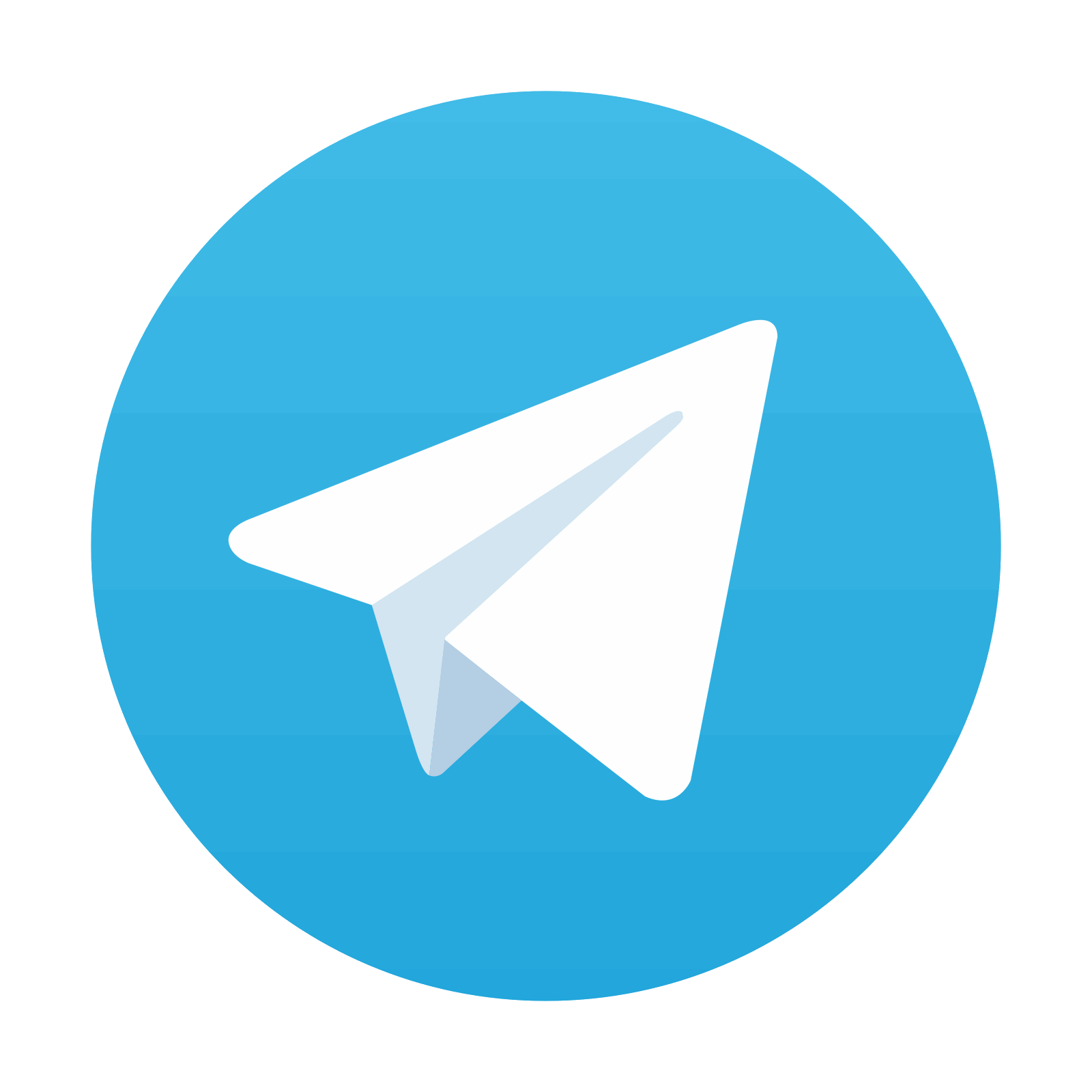
Stay updated, free articles. Join our Telegram channel

Full access? Get Clinical Tree
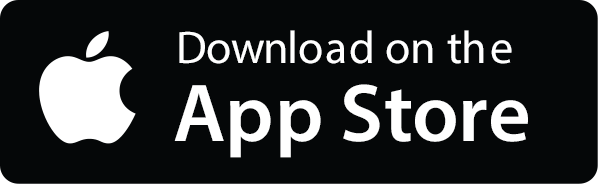
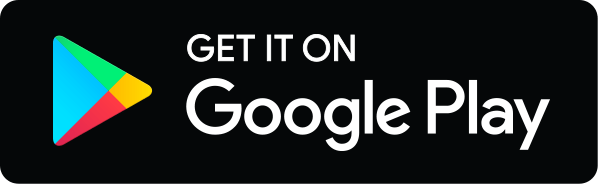