Short trunk
Brachyolmia spondylodysplasia
Short appendicular skeleton
Acromelic (short hand)
Acromelic dysplasia
Mesomelic (short forearm)
Dyschondrosteosis (Leri Weill)
Rhizomelic (short arm)
Achondroplasia and related disorders
Achondroplasia
Hypochondroplasia
Short trunk and limb
Spondyloepiphyseal dysplasia
Kniest dysplasia
The following broad groups (Table 20.2) are the conditions seen in a skeletal dysplasia clinic. Significant overlapping occurs within the group. Precise diagnosis is determined in consultation with an experienced geneticist.
Table 20.2
Relative common skeletal dysplasia groups
FGFR3 mutation |
Hypochondroplasia, achondroplasia, SADDAN (severe achondroplasia, developmental delay, acanthosis nigricans), thanatophoric dysplasia |
Metatropic dysplasia group and SMED |
Diastrophic dysplasia |
Type 2 collagenopathies |
SED congenita, SEMD, Kniest dysplasia |
Pseudoachondroplasia |
Multiple epiphyseal dysplasia |
Metaphyseal dysplasia |
Cartilage hair hypoplasia (McKusick), Schmidt, Jansen |
Chondrodysplasia punctata |
Dysostosis multiplex group |
Mucopolysaccharidoses |
Mesomelic dysplasia |
Leri–Weil dyschondrosteosis |
Dysplasia with predominant membrane bone involvement |
Cleidocranial dysplasia |
Bent-bone dysplasia group |
Campomelic dysplasia |
20.3 Spinal Problems in Skeletal Dysplasia
Spinal problems in skeletal dysplasia include instability, sagittal and coronal deformity, and stenosis. Some deformities are transient, for example, thoracolumbar kyphosis in achondroplasia which typically resolves at onset of ambulation and cervical kyphosis in diastrophic dysplasia. Cervical instability and stenosis in mucopolysaccharidoses is progressive that require early recognition and intervention to prevent or reverse neurological deterioration.
20.3.1 Base of Skull Abnormalities
Achondroplasia is a rhizomelic, disproportionate short statue with frontal bossing and mid-facial hypoplasia. In early life, they are at risk for hydrocephalus. Symptoms include irritability, lethargy, and vomiting. Head circumference measurement should be performed in reference to the normative chart of people with achondroplasia. Any upward crossing of the percentile would warrant further investigation with magnetic resonance imaging (MRI) scan of the brain. The defective endochondral ossification in patients with achondroplasia from the FGFR-3 mutation and early closure of synchondroses leads to foramen magnum and jugular foramen stenosis. It is thought that diminished jugular return due to jugular foramen stenosis results in hydrocephalus [2]. With growth, the risk diminished significantly. Management includes a ventriculoperitoneal shunt.
Platybasia literally means flattening of the skull base. It has been reported in patients with Kniest dysplasia [3] and cleidocranial dysostosis [4]. The sagittal relationship between the anterior and posterior fossa at the skull base (as measured by the base of skull angle, normal range <143°) pathologically widen. Indirectly, the relationship of the posterior fossa with upper cervical spine is altered as measured by clivus-canal angle (also known as craniovertebral angle, normal range 150–180°). Basilar invagination can ensue with the odontoid impinging on the ventral aspect of the cervicomedullary junction. In patients with cleidocranial dysostosis, the skull base angle is more likely to be wider than the normal population, possibly related to the abnormal flexure of the clivus, a midline structure that is malformed in conjunction with the clavicle and pubis [5].
20.3.2 Atlantoaxial-Occipital Complex Abnormalities
20.3.2.1 Developmental Anatomy
The upper cervical spine forms differently from the subaxial spine embryologically and is closely linked to the occiput [6]. The C1 atlas has three primary ossification centers (anterior atlas, arch, and two lateral masses). The closure of posterior synchondroses occurs at 3–5 years. An opening at the cartilaginous cleft can be found prior to the closure of the neural arch [7]. The closure of neurosynchondroses of the anterior atlas arch and the two lateral masses achieved by 5–7 years old [8]. The spinal canal area at C1 expands rapidly in the first 3 years of life with no further significant expansion beyond 6 years of age [9].
C2 has five primary ossification centers (two lateral masses, centrum, odontoid dens that is formed from two vertically aligned columns). C2 has secondary ossification centers that are located at the tip of odontoid, the roof and the base of the centrum, and the inferior ring apophysis. The atlantoaxial column is developed from separate embryological entities named X, Y, and Z. They become chondrum terminale, odontoid dens, and centrum of C2, respectively. Failure of fusion of XY and Z by ages of 5–7 results in an osodontoideum [10]. Failure of fusion of X and YZ by age of 12 results in an ossiculum terminale. The spinal canal expands rapidly in diameter prior to closure of the neurosynchondroses from birth to 8-year-old, but attains significant width by 2 years [11].
20.3.2.2 Foramen Magnum Stenosis and Cervical Stenosis
Achondroplasia classically has foramen magnum stenosis and cervical stenosis both in the upper and subaxial cervical regions. The symptoms may be subtle. The symptoms and signs include developmental delay, central apnea, neurological signs (hypotonia, hyperreflexia, clonus, hemiplegia, tetraplegia), or a combination of the above. Risk of sudden death is high when the stenosis is undetected [12]. Similar to the hydrocephalus, the risk is highest in the first 2 years of life and diminishes with the expansion of the spinal canal. In the suspected case, sleep study (central apnea) and MRI (Fig. 20.1) help to clarify the situation. Foramen magnum decompression is indicated in symptomatic patients with documented stenosis.
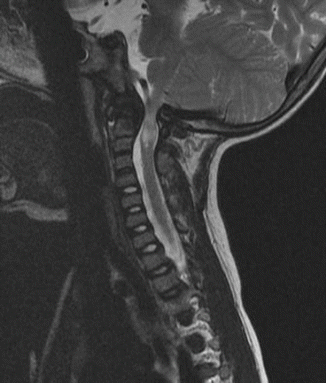
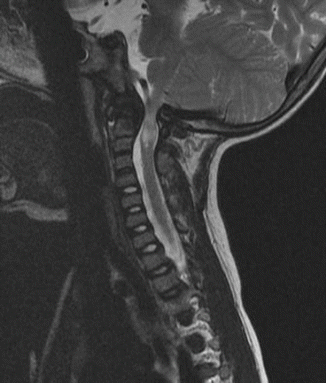
Fig. 20.1
Foramen magnum stenosis in achondroplasia. The MRI showed significant cervical magnum stenosis with cervical myelopathy in a patient with achondroplasia
20.3.2.3 Occipitalization of C1 and Pro-Atlas
C1 abnormalities are closely linked with occipital development. Failure of segmentation at the occipital sclerotome 4 and cervical sclerotome 1 result in occipitalization of C1 and potential basilar invagination. Occipitalization is reported in patients with skeletal dysplasia such as Goldenhar syndrome and Russell-Silver syndrome [13].
20.3.2.4 Upper Cervical Spine Instability
Upper cervical spinal instability includes occipito-cervical instability (O–C1) and/or atlantoaxial instability (C1–C2). This should be suspected in all cases of skeletal dysplasia with the exception of achondroplasia. The spinal instability may result in cervical myelopathy. The clinical manifestation ranges from asymptomatic to tetraparesis. In older children, the earliest symptom may be gradual loss of physical endurance. In toddler and younger children, gross and fine motor developmental delay can occur.
O–C1 stability is determined by ligamentous strength. Uniquely, there is lack of an intervertebral disk at this segment. The bony articulation of the atlas and occipital condyles are shallow and broad with a relatively lax capsule to allow motion. The cord-like alar and apical ligaments arise from the odontoid to the anteromedial aspects of the occipital condyles, and the tectorial ligament which is a continuation of the posterior longitudinal ligament to basion, are the most important internal ligaments at this joint. Instability at this joint is thought to be rare and includes Kniest dysplasia which is a type 2 collagenopathy [14].
C1–C2 stability depends on combinations of bony and ligamentous restraints. It is thought that the instability and associated delayed in ossification often seen in skeletal dysplasia result in odontoid abnormalities that ranges from odontoid aplasia, hypoplasia, to os odontoideum [15]. Failure of closure at the dentocentral synchondroses by 7 years results in os odontoideum. The line of separation may be caudad or cephalad to the superior articular facets of the axis (C2). This is in contrast to traumatic cause of dentocentral separation where the separation is caudad to the superior articular facets of the axis. The instability may result in delay or failure of ossification of chondrum terminale and anterior arch of the atlas. Dentocentral separation has been reported in chondrodysplasia punctata [16].
Abnormal appearance of the odontoid radiographically can be an indication of potential underlying upper cervical instability. However, not all abnormal odontoid morphology leads to instability. Skeletal dysplasias associated with odontoid abnormalities include pseudoachondroplasia, MPS, Cartilage-Hair dysplasia, diastrophic dysplasia, metatropic dysplasia, Larsen syndrome, and type 2 collagenopathy (SED, Kniest Dysplasia). Congenital nasopharyngeal abnormalities such as cleft palate may be associated with upper cervical spine abnormalities such as that is seen type 2 collagenopathy [14].
In atlantoaxial instability, there is either posterior or anterior subluxation of C1 on C2. Posterior subluxation of C1/C2 occurs when the atlas overrides the centrum of axis. This is a more uncommon phenomenon due to the odontoid process acting as a posterior constraint; however if there is odontoid aplasia (uncommon) or osodontoideum, there is mobility. Anterior subluxation can be due to odontoid abnormalities, ligamentous laxity, or absence of ligaments. In a lordotic cervical spine, a fixed posterior subluxation is probably more tolerable than an immobile anterior subluxation.
Cervical spine instability may not cause symptomatic spinal cord compression. In a normal C1 spinal canal, Steel’s rule of third dictates that one-third of the space is occupied by dens, one-third by spinal cord, and one-third is a reserve space that may be sufficient to buffer the instability created by a pathology. In Down syndrome for instance, symptomatic atlantoaxial instability is only present in 18 % of the patients with atlantoaxial instability [17]. Significant atlantoaxial instability (ADI > 8 mm) without symptoms is said to be an absolute indication for surgery while moderate atlantoaxial instability (ADI 4–8 mm) without symptoms is a relative indication for surgery. The authors do not agree. The most important factor is spinal cord compression and instability without spinal cord compression with a capacious canal does not require surgical management.
The surgeon must be aware of the concomitant presence of extradural impingement (see following section) or an abnormally small spinal canal at C1 which may be seen in spondyloepiphyseal dysplasia congenita [18] or metatrophic dysplasia [19]) (Fig. 20.2). Laminectomy of C1 may be necessary to create extra room to accommodate the spinal cord [18, 19]. In a detailed dynamic CT myelography study of patients with Type IV mucopolysaccharidoses, the source of impingement of the spinal cord was shown to arise from the abnormally thick posterior neural arch globally or at the unossified posterior neurosynchondroses focally [15]. It is our experience that cervical myelopathy presents earlier in patients with spondyloepiphyseal dysplasia congenita compared to patients with Morquio syndrome.
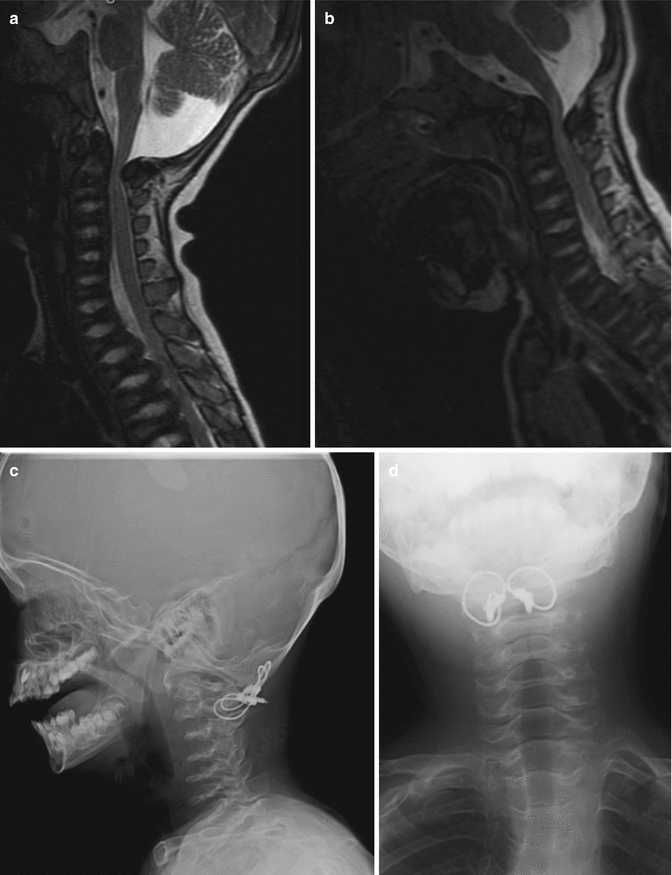
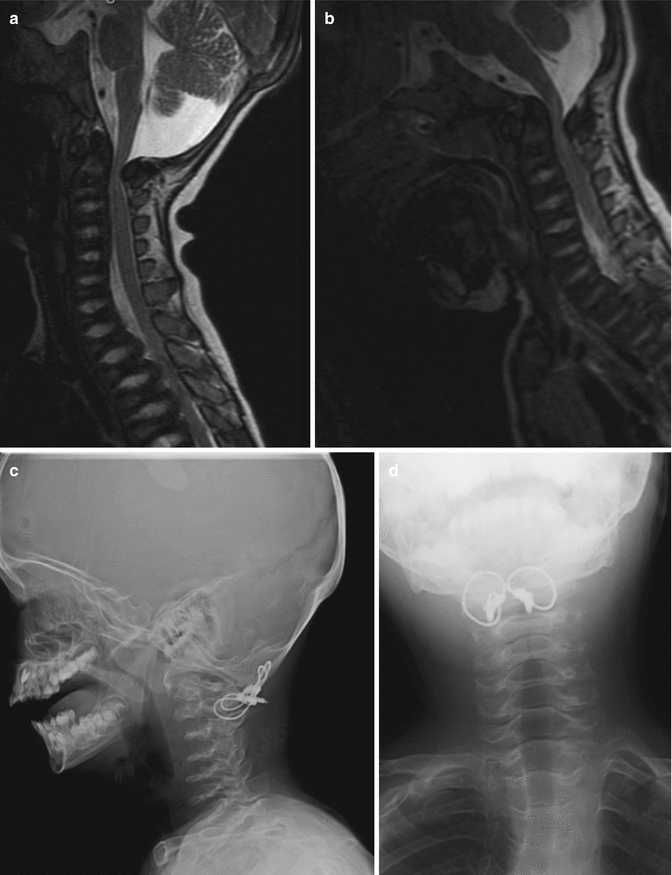
Fig. 20.2
C1 stenosis without instability in metatropic dysplasia. MRI views in flexion (a) and extension (b). C1 laminectomy was performed. Stabilization was achieved with posterior O–C2 arthrodesis using autologous bone graft and titanium cable. (c) Cervical X-ray lateral view and (d) cervical X-ray AP view postfixation
20.3.2.5 Abnormal Extradural Impingement
Extradural impingement of the spinal cord may be observed in mucopolysaccharidoses (Fig. 20.3). This is almost universal in mucopolysaccharidoses type IVa (Morquio syndrome) and to a lesser extent in type IH (Hurler syndrome) and type VI (Maroteux-Lamy syndrome). The glycosaminoglycan accumulation intracellularly results in mechanically incompetent cartilaginous tissue and ligamentous tissue that encourages reactive tissue formation. In cases where biopsy was performed via trans-oral approach, reactive tissue composed of fibrocartilaginous tissue accumulated extradurally without evidence of meningeal involvement [15]. It was also noted that the reactive tissue could extend caudadly to the dorsal aspect of the centrum of axis (C2). Extension to C3 and C4 is possible.
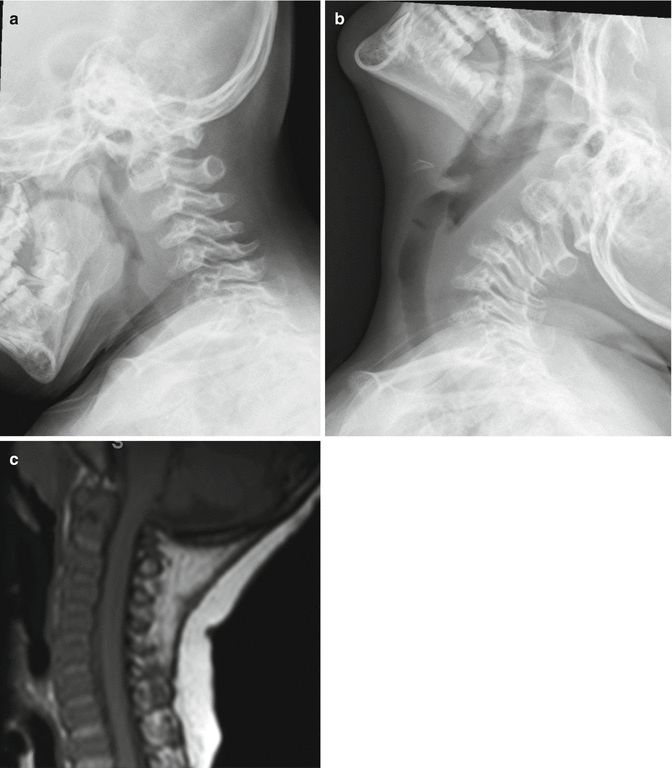
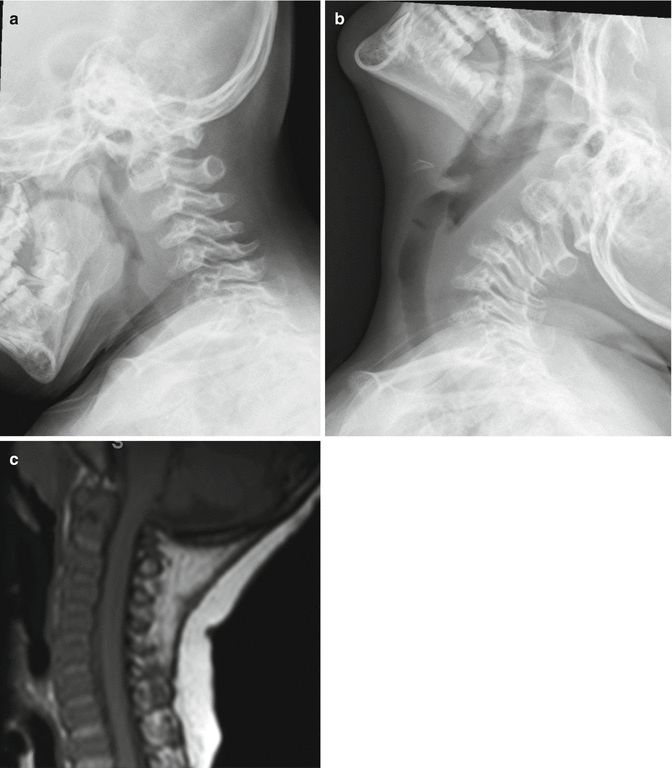
Fig. 20.3
Lateral cervical radiographs showing C1–C2 cervical instability in Morquio syndrome. SAC decreased in flexion (a) from extension (b). Extradural mass shown cranial to the hypoplastic odontoid process on T1 MRI cervical spine (c)
20.3.2.6 Torticollis: Atlantoaxial Rotatory Fixation/Subluxation/Dislocation
In patients with metatropic dysplasia, torticollis may occur as part of a possible mechanism to protect the airway [19]. Patients present with torticollis in extension. This can develop postoperatively in patients who had C1–C2 decompression without fusion or when fusion fails to occur. Patients may have torticollis secondary to a unilateral lateral mass defect rather than ligamentous rotational instability [20].
It is postulated that recurrent upper viral respiratory infection involving Waldeyer’s tonsillar ring seen in the Griesel’s syndrome may potentially predispose the at-risk patients with cleft palate or recurrent otitis media to atlantoaxial rotational subluxation. The hyperemic tissue at the posterior wall of nasopharyngeal space irritates the atlantoaxial joint asymmetrically resulting in instability. Atlantoaxial rotational subluxation after otoplastic surgery has been reported in patients without skeletal dysplasia (21). The diagnosis is confirmed by dynamic CT scan.
20.3.2.7 Radiological Signs of Upper Cervical Spine Instability
Radiological investigation using plain radiography may underestimate the underlying cervical instability. The occipitocervical junction is difficult to visualize. The immature bone with delayed ossification centers at various stages and abnormalities of the odontoid process compound the issue. When in doubt, dynamic MRI of the cervical spine should be performed [3] (Fig. 20.4). In very young children, this entails general anesthesia. MRI is done in neutral position first. Compression seen at this position may obviate the need for further dynamic positioning.
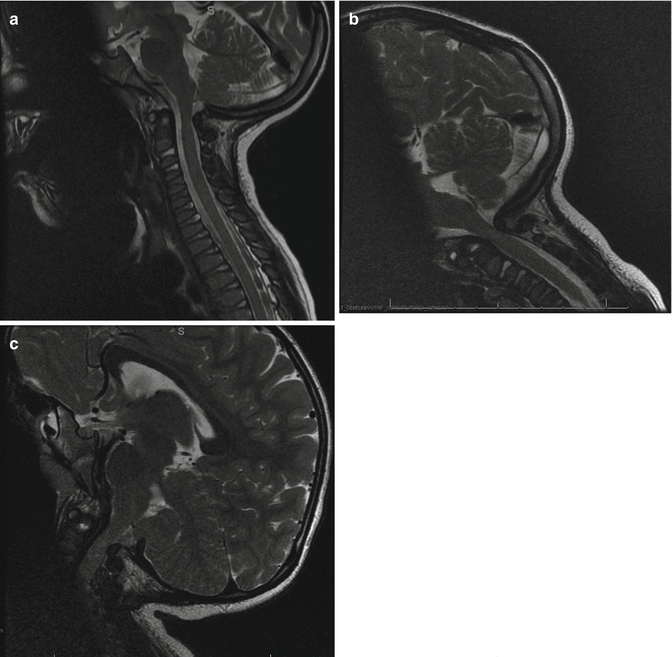
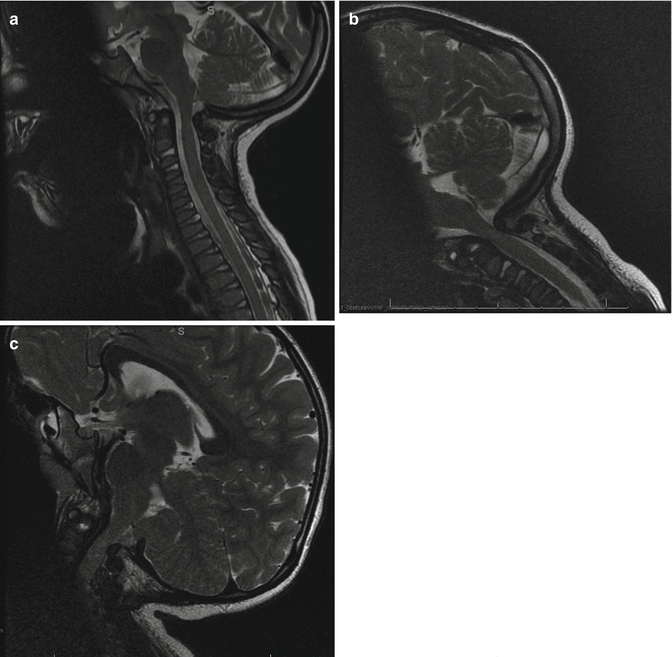
Fig. 20.4
Dynamic MRI. Space available for cord (SAC) in neutral position of the MRI (a). The stenosis is most severe in flexion view (b) and improved with extension (c)
The standard measurement for O/C1 horizontal instability includes Power ratio, Basion-Dental interval, or Wiesel-Rothman method. The latter is the easiest to measure.
Vertical instability that results in basilar invagination is ascertained by Mc Rae’s line, Chamberlain’s line, McGregor’s line, or Wachenheim’s line. C1 involvement from occipital condylar hypoplasia, for example, is determined by Kaufman’s technique.
C1/C2 horizontal instability is measured by anterior atlantoaxial distance, an indirect measure, or posterior atlantoaxial distance (also known as space available for cord [SAC]).
20.3.2.8 Surgical Options
Cervical arthrodesis at C1–C2 include Gallie’s technique, Brooks-Jenkins’s technique, Sontag modification of Gallie’s technique, Magerl’s transarticular screw [21], and Harm’s modification of Goel’s technique [22]. Gallie’s technique has poor rotational stability at the C1–C2 joint with a high rate of nonunion. Screw-based techniques (Magerl’s and Harm’s) are effective and can be used in young patients but careful preoperative evaluation of the vascular anatomy needs to be done (Fig. 20.5).
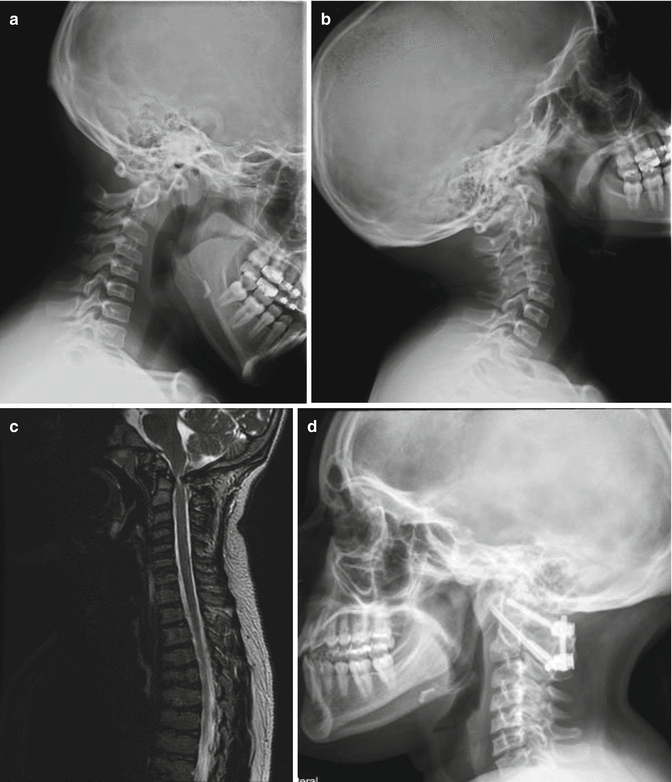
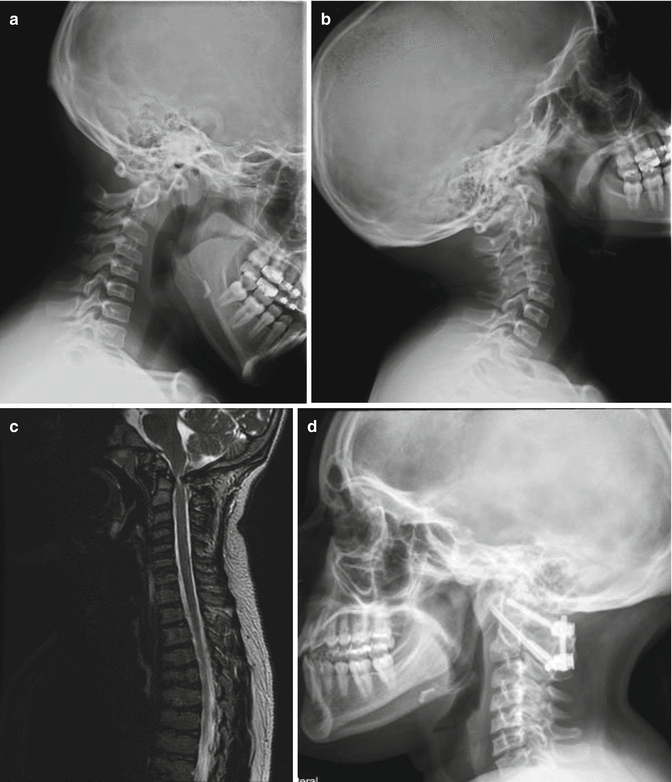
Fig. 20.5
SEDC with C1–C2 instability in flexion (a) and extension (b) views. MRI (c) shows cervical stenosis and myelomalacia. C1–C2 decompression, instrumentation, and fusion were done as shown in (d)
The Brooks-Jenkins’s technique [23] and the Sontag modification of Gallie’s technique [24] are effective wire-based techniques for C1–C2 arthrodesis. These two techniques are indicated for horizontal or vertical instability without the need for C1 ring decompression. C1 ring decompression is required if there is compression in a reduced position or if it is secondary to an irreducible subluxation. Fixation is ideally achieved with the Magerl or Harms technique but in young children this can be difficult especially if extension is needed to the occiput which may be needed in patients with spondyloepiphyseal dysplasia congenita, Morquio syndrome, or metatropic dysplasia.
To achieve occiput-cervical fusion after decompression, we used a modified occipital-based technique with a fashioned autologous iliac bone graft notched between O and C2 using cable (Fig. 20.2). Immobilization was performed using halo-vest postoperatively. No nonunion is reported [22]. Other techniques for O to C2 fusion include the use of rib graft and wire-based fixation of a fashioned iliac crest bone graft. Occipito-cervical fixation is often too bulky in young children.
20.3.3 Subaxial Cervical Spine Abnormalities
20.3.3.1 Developmental Anatomy
The subaxial cervical spine develops differently from the upper cervical spine. The subaxial cervical spine derives from sclerotomes C3 to C8. Primary ossification centers of the centrum first appear in the lower cervical/upper thoracic region and progresses caudad-cranially to C3. The centrum unites with the neural arch by 3 years of age. They are usually wedge-shaped with some loss of lordosis until about the age of 8–10-year-old. Ossification of the apophyseal rings appears at 10–12 years and the rings are fused by skeletal maturity.
20.3.3.2 Subaxial Cervical Spine Kyphosis and Stenosis
Subaxial cervical spine kyphosis should be screened for in patients with diastrophic dysplasia and Larsen syndrome. Other skeletal dysplasias with cervical spine kyphosis include Kniest dysplasia, chondrodysplasia punctata, and campomelic dysplasia [19, 25]. Rarely, Morquio syndrome may present with cervical kyphosis [26].
In mild cervical kyphosis, spinal alignment is altered without neurological consequence. With progression, the cervical kyphosis may result in instability with ventral cord impingement in flexion and relieved with extension. A significant deformity causes cervical stenosis in both flexion and extension. The progression of the deformity depends on the individual skeletal dysplasia and includes the magnitude of the deformity at presentation, the loss of anterior column support, the failure of facet and capsular restraints, associated posterior element deficiency, and the loss of the posterior tension band.
In diastrophic dysplasia, one-quarter of the patients have cervical kyphosis at birth. The kyphosis has its apex at C3 to C4, less commonly at C5 (Fig. 20.6). With the preservation of lordosis at the caudad level, a S-shaped or swan-neck appearance is evident. The vertebral body at the apex is hypoplastic resulting in triangular or round shape, also termed “loss of four corner” [27]. Concurrent spinal bifida occulta is often present from C3 to upper thoracic spine [28]. Most of the cases resolved spontaneously by the age of 6 years. In the largest series to date [27], cervical kyphosis of more than 60° (Fig. 20.7) at presentation had poor prognosis with either progression of the kyphosis or respiratory failure from associated severe tracheal and bronchiomalacia in early life.
