11 Technical Innovations and Robotic Skull Base Surgery
David W. Kennedy and John M. Lee
Introduction
Intranasal surgery has undergone tremendous change and evolution throughout the last century. Along with an improved understanding of anatomy and physiology, technical advances in visualization, imaging, and instrumentation have provided surgeons with better access to treat a variety of paranasal sinus diseases. As more experience and skill was gained, the boundaries of endoscopic sinus surgery expanded to the skull base and intracranial cavity. This chapter highlights the key technical innovations that have provided the stimulus and tools for this exciting and growing field.
History
The origins of nasal endoscopy can be traced back to 1901 when Hirschmann used modified cystoscopes to examine the sinonasal cavity.1,2 These cystoscopes consisted of a series of glass lenses interspersed by air. In 1902, Reichert was then credited with performing the first endoscopic sinus surgery using a 7-mm endoscope through oroantral fistulas to perform maxillary sinus manipulations.3 It was not until 1925 that Maltz promoted diagnostic endoscopy of the sinonasal cavity and coined the term “sinoscopy.”1 However, issues with illumination and depth of field prevented its widespread acceptance and use. Perhaps the most significant technical innovation in the history of endonasal surgery was the development of the rod optic endoscopic system by Harold H. Hopkins in the 1960s. By using glass spacers in between a series of glass lenses, optical image quality improved dramatically with better resolution, wider depth of field, and a sixfold increase in light intensity. This rod-optic system was then introduced to Karl Storz, whose medical instruments company refined what would be become the modern endoscope.4,5
Using the improved endoscope, Messerklinger studied nasal mucociliary clearance in fresh cadavers and wrote a book on clinical endoscopic diagnosis.6 It was not long before Messerklinger, Draf, Wigand, and others began performing endoscopic nasal procedures.7 After visiting these surgeons Kennedy introduced endoscopy to North America, validated the principles in a rabbit model, further developing the techniques and introducing the term functional endoscopic sinus surgery (FESS). In conjunction with Heinz Stammberger, the first-ever course in functional endoscopic sinus surgery was taught at Johns Hopkins University in 1985.8 Over the last 20-plus years, endoscopic sinus surgery has become the standard of care for chronic sinus disease refractory to medical therapy. As further experience and skill was gained, both otolaryngologists and selected neurosurgeons recognized the potential for the endoscope in skull base and intracranial surgery. Although the first hesitant steps in skull base surgery were made in the 1980s, one decade later multiple institutions had begun popularizing the use of endoscopy in pituitary surgery, evolving from a combined microscopic and endoscopic technique to an exclusively endoscopic transsphenoidal approach.9–13 As techniques and technologies have evolved, the boundaries of endoscopic transnasal surgery have expanded to the anterior skull base and parasellar and paraclival regions. Described below are some of the most important innovations to have occurred that enabled this dramatic evolution.
Visualization
Since the invention of the rod-optic system, there has been more refinement rather than evolution of the endoscope itself. The large variety of zero-degree and angled endoscopes in both 4- and 2.7-mm diameters provide the endoscopic surgeon with numerous options to navigate the paranasal sinus cavity. However, perhaps the most dramatic evolution has occurred in the development of the wide-angle, 45-degree endoscope, which enables both direct forward vision and a deflected angle of view close to that achieved by a 70-degree endoscope, while at the same time providing improved illumination. Such endoscopes make it easier for surgeons, particularly those such as neurosurgeons who are less familiar with passing endoscopes intranasally, to operate under endoscopic control. One drawback of the endoscope is the two-dimensional mono-ocular image that is created. This has engendered some reservation among neurosurgeons who are familiar with the three-dimensional (3D) binocular view of the microscope. Multiple successful and innovative attempts have been made to produce 3D images with either dualchannel endoscopes or dual cameras, and even computer-generated endoscopic 3D views. Excellent pictures can be generated as a result of the dual-channel endoscope, and this technology has now been incorporated as the standard visualization arm in robotic surgery.14 However, technical issues such as the larger endoscope size, the necessity for special goggles or headsets, and especially an inability to appropriately rotate a 3D image while turning an angled endoscope have prevented its adoption in standard transnasal endoscopic surgery.15 Although the latter is not an issue with single-channel 3D endoscope technology, the 3D quality is inferior, and, as a result, it has not proven significantly advantageous at this point in time.
One area that has evolved dramatically in visualization is the camera technology used to project images from the endoscope onto a monitor. The original tube cameras had poor light sensitivity, especially in a field with any blood and generated poor color rendition. This often meant that the surgeon would be dependent on looking through the endoscope to operate safely. However, these concerns were subsequently addressed with light-sensitive charge-coupled device (CCD) cameras that produce improved images, and more recently with high-definition cameras and monitors, providing an excellent image so that endoscopic surgery can now routinely be performed from a monitor with exceptional detail. In addition to an improved ability to teach endoscopic techniques, operating from a screen also facilitates two-surgeon and potentially four-hand techniques, which are useful in skull base surgery (Fig. 11.1).
Fig. 11.1 Two-person, four-hand technique for endoscopic skull base surgery.
Through-Cutting Instruments and Microdebriders
Although early endoscopic sinus surgery decreased the extent of surgery compared with traditional external approaches or headlight transnasal techniques, early instruments largely consisted of Blakesley forceps, which tended to strip mucosa and create significant postoperative scarring and persistent inflammation.7,16 Drawing from orthopedic surgery, fine through-cutting instruments were developed for intranasal use. Both straight and 45-degree through-biting instruments enabled surgeons to cut through both bone and mucosa while preventing inadvertent stripping of nasal cavity epithelium. These instruments have also proved to be especially important when removing bony partitions along the skull base and also within the frontal recess and sphenoid sinus.17 Blunt manipulation of bony septations adjacent to the skull base or internal carotid artery can lead to disastrous outcomes if unpredictable bony breaks are created. More recently, hand instrumentation has begun to be developed for transnasal skull base and intracranial surgery. This surgery requires very fine and somewhat longer instrumentation and atraumatic suction devices. In addition, the tip of the instrument should ideally not move when the instrument is activated, as may happen with some of the typical forceps and scissors designed for sinus surgery. Continued improvement of the range of instrumentation designed for transnasal craniectomy is anticipated.
The concept of through-cutting instruments has also evolved via powered instrumentation. Microdebriders were originally developed for small-joint arthroscopy and joint removal. In 1996, Setliff18 and Parsons19 introduced this device for nasal use, thus facilitating sinus tissue and bone removal. Subsequent improvements in blade and power technology, as well as the addition of angled blades have significantly revolutionized our surgical techniques (Fig. 11.2). The combination of sharp tissue removal and concurrent suction allow for excellent visualization while reducing the risk of mucosal stripping. In addition to removal of benign inflammatory disease, this technology has also been a useful adjunct in the initial debulking of certain tumors such as inverted papillomas and other benign and malignant lesions, thus enabling the sites of attachment to be carefully identified. However, beyond the bony confines of the sinonasal cavity, the potential for orbital, intracranial, and vascular injury limits the safety and utility of the microdebrider.
Electrical Devices
Early in endoscopic sinus surgery, it was believed that lasers would be an excellent tool for the removal of sinonasaal tissue and for providing hemosatasis control. Although a wide variety of lasers were tried, including KTP (potassium titanyl phosphate) and YAG (yttrium-aluminum-garnet), it soon became apparent that collateral damage from lasers was a significant problem, with increased scarring and long healing times.20,21 However, there were a couple of key innovations that have since evolved from the use of lasers. Schuman and Pineyro22 modified the YAG laser technique by using constant warm isotonic irrigation. Their observation of warm water irrigation causing nasal vasoconstriction is a concept that is still often employed today, especially in a very vascular surgical field.23 Second, the pulsed holmium:YAG laser suggested by Shapshay et al24 often resulted in blood splashing back onto the lens each time the laser was fired. Consequently, Shapshay et al. developed the precursor device that would later be modified into the Endo-Scrub pump and sheath, which irrigates and cleans the tip of the endoscope (Fig. 11.3).24 This has become an important addition to endoscopic equipment, as it facilitates good visualization despite a bloody operative field.
Fig. 11.2 Powered instruments: Gyrus ACMI (Southborough, MA) microdebriders.
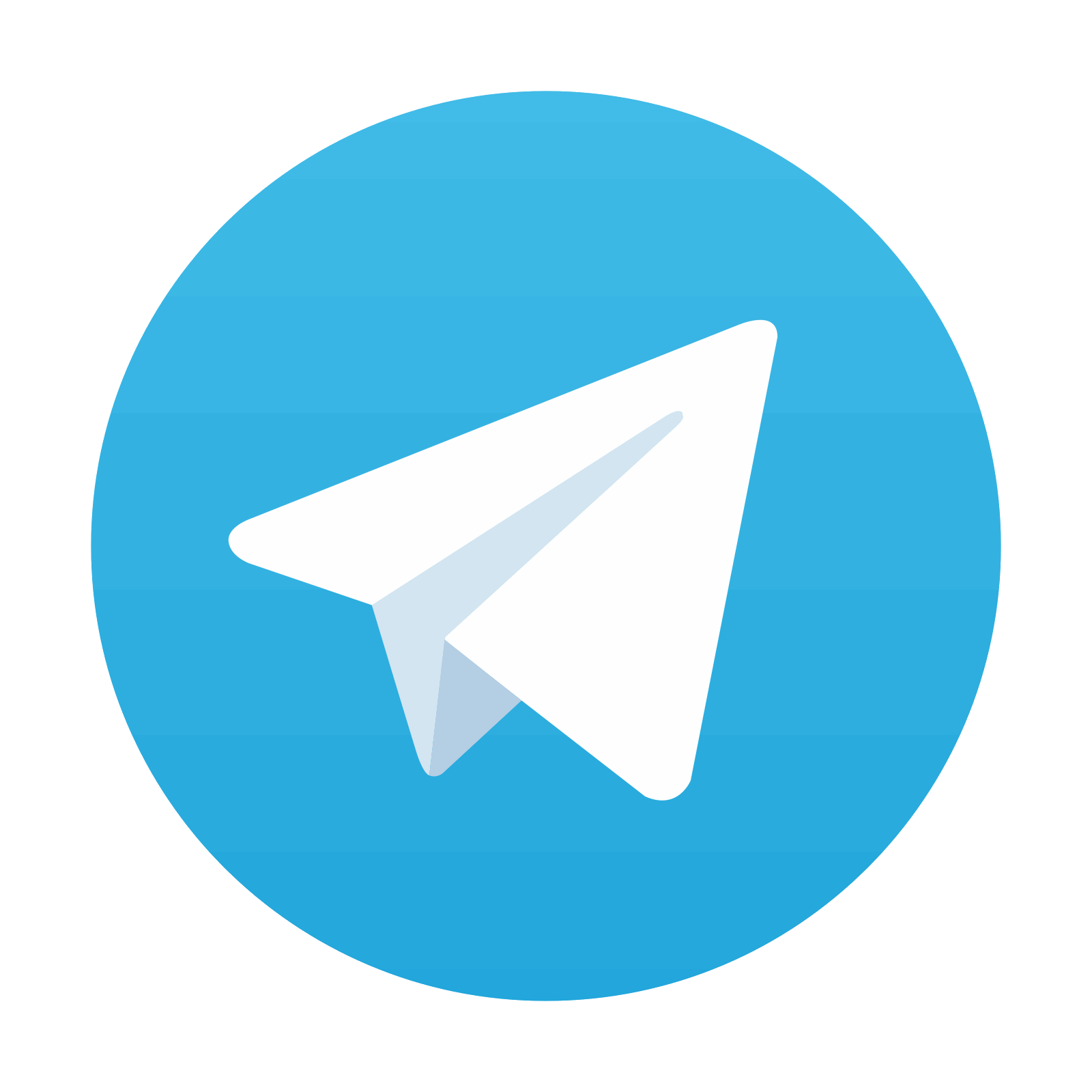