Chapter 17 Case This 40-year-old otherwise healthy woman came to medical attention with recurrent episodes of numbness in her left hand. Digital subtraction angiography revealed a 1.5-cm right parietal arteriovenous malformation fed by distal branches of the middle cerebral artery with superficial drainage into the superior sagittal sinus. A T1-weighted axial magnetic resonance image with contrast confirmed that this lesion was within millimeters of, but posterior to, her primary somatosensory cortex. Participants Microsurgery for Small Arteriovenous Malformations: Bradley A. Gross and Rose Du Gamma Knife Radiosurgery for Arteriovenous Malformations: Mark E. Linskey Proton Beam Stereotactic Radiosurgery for Arteriovenous Malformations: Jay Loeffler and Pankaj K. Agarwalla Moderators: The Treatment of Arteriovenous Malformations in Eloquent Areas: Ramsey Ashour and Jacques J. Morcos Cerebral arteriovenous malformations (AVMs) are a considerable source of morbidity and mortality in neurosurgical patients, often as a consequence of hemorrhage but also as a result of debilitating seizures and neurologic deficits.1–4 Management options include observation, microsurgical resection, radiosurgery, embolization (often adjunctive or palliative), or a combination of these approaches (Table 17.1). Observation is a consideration for large AVMs in eloquent locations (high grade), particularly if they are asymptomatic or discovered in older patients. Small AVMs (less than 3 cm), particularly if symptomatic or certainly if they have previously hemorrhaged, are ubiquitously considered for intervention. Microsurgical resection is the time-honored therapeutic modality in the treatment of AVMs, providing immediate and often definitive therapy. In reviewing the natural history, microsurgical, and radiosurgical results for small AVMs, we show that microsurgery should be considered first, unless the lesion is in a surgically inaccessible, eloquent location or the patient is medically unfit for surgery. Although most patients with AVMs come to attention because of hemorrhage, 20% present with seizures, with an annual development rate of de novo seizures of 1%.1 Factors associated with a greater risk of epileptogenesis from AVMs include younger age, a cortical or temporal location (or both), and a larger size. Approximately 7 to 10% of patients with AVMs present with nonhemorrhagic focal neurologic deficits, in some cases attributable to local steal phenomena.1–4 Reviewing both modern and older natural history studies reveals a consistent overall annual hemorrhage rate of 2 to 4% for AVMs.1–4 More recent reports have consistently shown an increased risk of hemorrhage among patients with a prior hemorrhage, with overall annual re-bleed rates of 3 to 7%.2–4 Early re-bleed rates in the first year range from 6% to as high as 15% in some studies.3 Associated aneurysms, deep venous drainage, and a deep location were often associated with further elevated hemorrhage risks, independent of hemorrhagic presentation.2–4 Although a small AVM size is not consistently noted among these studies, it has also been proposed as a risk factor for hemorrhage, potentially as a result of increased feeding artery pressures.5 Given the risk of rehemorrhage, we recommend treatment of all small AVMs that have bled. To potentially mitigate and prevent the progression of seizures or focal neurologic deficits, we also recommend the treatment of symptomatic small AVMs. Unruptured, asymptomatic small AVMs should also be treated given the lifetime risk of hemorrhage and the development of focal deficits or seizures, particularly in younger patients. Microsurgical resection of AVMs is the time-honored treatment modality that affords an immediate cure in the vast majority of patients. The associated risk is up-front, with outcomes generally improving as follow-up time accumulates. The Spetzler–Martin grading scale, used to predict surgical risk, underscores the importance of size as a factor influencing the difficulty of resection.6 In essence, any small AVM would be deemed operable by this scheme given that all AVMs of less than 3 cm would receive a grade of I to III. Nevertheless, an AVM in an eloquent locale such as the motor cortex, internal capsule, or brainstem, without a corridor of access, regardless of size, may be deemed inoperable. In addition to anatomic corridors of access, a hematoma may provide access to an eloquent AVM and even facilitate dissection by separating the nidus from the brain. Thus, in addition to factors such as eloquence and deep venous drainage evaluated by the Spetzler–Martin scale, the AVM rupture status and the patient’s neurologic status should be considered. Although both may redefine the significance of an AVM in an eloquent locale, a prior hemorrhage also indicates surgical intervention given the greater risk of rehemorrhage, particularly in the first year after rupture. On the other hand, an AVM with a significant perforator supply, a diffuse nidus, or both may be particularly more challenging from a surgical vantage point, in spite of the fact that these factors are not included in the Spetzler–Martin grading scale.7 Reviewing results from surgical series of small AVMs, we see exceedingly high obliteration rates (94–100%) with very limited surgical morbidity and mortality (0–3%) (Table 17.2).8–13 Lawton’s13 series reflects results from 35 grade III AVMs with only one death that was attributed to fulminant liver failure. In this series, Lawton illustrates that small grade III AVMs present the lowest surgical risk compared with moderate-sized (3–6 cm) AVMs with deep venous drainage or in an eloquent location. These exceptional results reinforce the fact that any small AVM, particularly if ruptured, should always be considered for microsurgical treatment first. Although small AVM size is associated with greater rates of AVM obliteration,14 the exceptional surgical results we illustrate in Table 17.2 reinforce the fact that radiosurgery remains a secondary option for small AVMs, reserved for those deemed to be of high operative risk. This category essentially includes patients with small AVMs in eloquent locales without a reasonable corridor of surgical access. In a group of 81 small AVMs treated with radiosurgery, the obliteration rate was 90%; however, nine patients (11%) suffered from AVM rupture after stereotactic radiosurgery (SRS), resulting in three deaths.15 Five patients also suffered adverse radiation effects (6%). Although most SRS series do not stratify results according to the size of the AVM, the University of Pittsburgh group recently published its experience with SRS of 217 grade I or grade II AVMs (primarily small AVMs).16 The overall obliteration rate was 58% at 3 years, 87% at 4 years, and 93% at 10 years. However the actual angiographically confirmed obliteration rates were 41% at 3 years, 66% at 4 years, and 83% at 10 years. Five patients (2%) suffered from transient radiation effects, two (1%) developed delayed cysts, and 13 (6%) suffered from hemorrhage after SRS. Six patients (3%) died of hemorrhage during the latency period. These results compare unfavorably to those for surgery of small AVMs. An evaluation of radiosurgical results is further limited by the methods used to report obliteration and the fact that complications accrue with time. The importance of confirming the obliteration of AVMs after microsurgery leads to consistent reporting of formal, angiographically confirmed obliteration rates. On the other hand, many radiosurgical reports inflate obliteration rates by using magnetic resonance imaging (MRI) in lieu of formal angiography to confirm obliteration. In fact, even patients with complete angiographic obliteration after SRS have been known to have delayed rebleeding.17 In addition to delayed hemorrhage and adverse radiation effects after SRS, delayed cyst formation, arteriopathy, and even de novo pseudoaneurysm development may occur (Fig. 17.1).15–18 Debilitating radiation necrosis may even require surgical resection.19 These events occur at overall rates proportional to the actual follow-up time. Unlike after successful microsurgical obliteration of AVMs, rupture after SRS is a cause of morbidity and mortality.15–18 Given the greater risk of re-rupture from ruptured AVMs, these AVMs should be managed through surgery unless they are embedded in eloquent tissue without any reasonably safe surgical corridor (e.g., the brainstem). Furthermore, associated aneurysms should be treated expeditiously and taken into account when considering SRS. In the report from the University of Pittsburgh team, the rate of bleeding of an AVM with an associated aneurysm after SRS was 28% at 5 years.16 Germane to the case at hand, seizure outcomes are generally better after surgery for AVMs as compared with SRS. In one study of 110 patients with epileptogenic AVMs, 81% of those undergoing surgery were free of disabling seizures at follow-up as opposed to only 43% of patients undergoing radiosurgery.20 Furthermore, from a lifestyle perspective, the ability to achieve an expedient cure of the AVM through microsurgery allows for the swifter alleviation of potentially debilitating seizures. Fig. 17.1a,b (a) A 53-year-old man with seizures underwent radiosurgical treatment of a 2.5-cm temporal arteriovenous malformation (AVM). Although the lesion was obliterated, this digital subtraction angiogram at 2.5 years’ follow-up shows de novo stenosis of a former middle cerebral feeding artery (arrow). (b) A 50-year-old man returned 14 years after radiosurgery for his callosal AVM with a devastating rupture of one of two de novo pericallosal pseudoaneurysms (arrows). Planning a strategy for AVMs requires a systematic approach that first evaluates their natural history followed by the potential treatment options and their attendant risks and benefits. The malignant natural history of ruptured AVMs warrants treatment. We strongly believe that surgery should be used to avoid the risk of hemorrhage in the latency period. Although unruptured AVMs have a relatively more benign natural history, they continue to pre sent a risk of hemorrhage as well as morbidity from potential neurologic deficits or seizures. We believe that symptomatic small AVMs should be treated with microsurgery, given the attendant risk of hemorrhage and continued or even worsening morbidity. Surgical results are clearly superior to those of radiosurgery for small AVMs, except for lesions embedded in eloquent cortex without safe surgical access. The ARUBA study (A Randomized Trial of Unruptured Brain AVMs) is a prospectively designed trial aimed at comparing observation with outcomes after surgery for unruptured AVMs.21 The early pilot paper will likely show worse outcomes in the surgical group given the up-front, early risks that this cohort must undertake. With sufficient follow-up, morbidity and mortality from hemorrhage will accumulate and eventually outweigh the risks of surgery as early postoperative deficits improve. Unfortunately, ample follow-up will require years, and we hope the study does not conclude early because of the expected better early outcomes in the observed cohort. The same concept applies to radiosurgery. As with observation, complications from radiosurgery accrue with time. The patient faces a cumulative, continued risk of hemorrhage, radiation necrosis, delayed cyst formation, and even arteriopathic complications with time (Fig. 17.1).15–18 These complications are at the expense of a chance, rather than a guarantee, of obliteration. Although significantly greater obliteration rates after microsurgery as compared with radiosurgery are not a subject of debate, we strongly believe that the cumulative side effects of radiosurgery clearly outweigh the up-front risks of microsurgery for small AVMs, as long as they are surgically accessible. We would offer microsurgery to the patient in this chapter’s case presentation. Given her young age and her symptoms, microsurgery provides a definitive means of early cure, potentially alleviating her symptoms and eliminating her lifetime risk of hemorrhage. Radiosurgery would provide a lower chance of cure at the expense of a latency period of hemorrhage and delayed adverse radiation events. This case represents a technical challenge for any treatment approach as well as an excellent platform for philosophical discussion. At less than 3 cm in size (< 1.5 cm in size with 1.75 cm3 in volume), with superficial venous drainage, and a location in a primary sensory strip, this lesion represents a Spetzler–Martin grade II AVM.6 The patient’s history suggests the possibility of simple partial sensory seizures for the mode of presentation. Neither the history nor MRI suggests any recent hemorrhage, which might potentially increase the short-term risk of AVM hemorrhage.4,22 The images provided are limited, but there does not appear to be evidence of restricted venous out-flow, an intranidal aneurysm, a draining varix, or a feeding artery aneurysm. The presence of any of these factors might be expected to increase the chances of the annual risk of rupture.23–27 According to actuarial data from the United States Social Security Administration in 2007, at age 40, this patient has a median life expectancy of an additional 44 years.28 With an annual hemorrhage rate for unruptured AVMs of 1 to 2.2% per year,29–31 this information translates into a generous lifetime risk estimate of AVM hemorrhage of 55%.32 Given a general per-hemorrhage mortality risk of 10%, with another 10% risk of permanent major neurologic morbidity,33 expectant observation with an acceptance of the consequences of the natural history, in our opinion, would not be a prudent recommendation. Several validating microsurgical studies of the Spetzler–Martin grading system suggest a 36% chance of any deficit, a 0 to 6% chance of a permanent disabling deficit, and a less than 1% mortality rate for microsurgical resection of this lesion in very experienced cerebrovascular microsurgical hands.6,34–36 There is evidence, however, that not all “eloquent” locations are equally eloquent when it comes to assessing the treatment risk. Precentral involvement is particularly susceptible to a permanent new deficit.37 Al-though this AVM is immediately postcentral in location, its draining vein is the vein of Trolard, which provides the dominant venous drainage for the precentral gyrus and primary motor strip. Any interruption in this drainage runs some risk of venous infarction of the primary motor strip. The lesion appears to have a single arterial feeder to a tight compact nidus, which potentially increases the chance of endovascular cure with complete and permanent obliteration of the nidus.38–41 However, periprocedural risks remain heavily weighted toward primary motor strip morbidity from either the arterial or venous side, which would portend a potentially significant permanent neurologic deficit. Indeed, at present, curative embolization of AVMs remains somewhat problematic, with utility better proven for preparatory microsurgery or SRS. Stereotactic radiosurgery is very feasible for this small AVM. Radiosurgery obliteration rates are dose-threshold dependent, with a predictive obliteration rate of more than 80% if 20 Gy or more can be delivered to the nidus margin.42 This small AVM could certainly tolerate 20 Gy to the margin from a dose-volume toxicity standpoint.42,43 Indeed, the predictive obliteration rate at 3 to 5 years is 90%.16 The main risks of SRS are AVM hemorrhage during the latency period of 3 to 5 years and temporary, symptomatic, adverse radiation effects. The risk of hemorrhage during latency is 9%32 (translating into a 0.9% mortality risk and an additional 0.9% risk of permanent neurologic morbidity33) over 5 years, plus those associated with a 10% risk of non-obliteration. The adverse radiation effects comprise a 20% risk of MRI-detectable signal change on the T2-weighted fluid-attenuated inversion recovery (FLAIR) images at 3 to 9 months after radiosurgery, of which less than 10% would be temporarily symptomatic. Assuming a 12-Gy volume of less than 5 cm3, the chance of a new, permanent neurologic deficit can be predicted to be less than 1 to 2%.43 With all these considerations in mind, we recognize that microsurgical excision, an attempt at curative embolization, and SRS are all reasonable options for treatment based on the individual patient’s view of the relative advantages and disadvantages and the patient’s life goals and priorities. We would likely advise SRS as the preferred and recommended approach. Stereotactic radiosurgery has been defined by the American Association of Neurological Surgeons, the Congress of Neurological Surgeons, and the American Society for Therapeutic Radiology and Oncology as typically “performed in a single session using a rigidly attached stereotactic guiding device, other immobilization technology and/or a stereotactic image guidance system, but can be performed in a limited number of sessions, up to a maximum of five.”44 For AVMs, SRS is optimally done in a single session. There are no good data to suggest multisession radiosurgery or fractionated radiotherapy to treat AVMs. Single-session radiosurgery can be done with any one of several different technologies: • The gamma knife (Elekta AB, Stockholm, Sweden) • The CyberKnife (Accuray, Inc., Sunnyvale, CA) • The Novalis system (BrainLAB AG, Feldkirchen, Germany) • The Varian Trilogy system (Varian Medical Systems, Inc., Palo Alto, CA) • The X-knife (Integra Life Sciences, Radionics Division, Plainsboro, NJ) • Charged particle devices (protons, helium, and carbon) The gamma knife is a cobalt 60 (60Co)-based system. The CyberKnife, the Novalis and Varian Trilogy systems, and the X-knife are linear accelerator-based, and charged particle systems require a cyclotron. As long as a minimum dose threshold is achieved, all SRS modalities have similar results. Certainly, gamma knife SRS has the largest portfolio of published evidence to support its use. Of the 1,145 articles documenting the use of radiosurgery for AVMs published in English and listed in the National Library of Medicine Medline database as of February 2012, 70.2% report the results of gamma knife SRS. Linear accelerator results account for 23.1% of publications (only 1.75% of publications involve the CyberKnife), whereas reports of the results of charged particle units account for 6.6% of peer- reviewed publications. In our center, we prefer the Leksell Perfexion Gamma Unit (Elkta AB, Stockholm, Sweden) for SRS. In this chapter’s presented case, we would use both catheter angiography and stereotactic MRI studies for targeting to eliminate veins from the target volume as well as optimize and limit the three-dimensional nidus definition. By limiting the nidus volume definition, we would minimize potential treatment morbidity from adverse radiation effects as well as maximize our safe minimal dose prescription. Multi-isocenter planning is the cornerstone for achieving three-dimensional conformality when treatment planning with gamma units. For this patient, we would anticipate a 90% chance of AVM obliteration 3 to 5 years after radiosurgery using our treatment strategy, with a 0.9% mortality risk and an additional 0.9% risk of permanent neurologic morbidity from hemorrhage during the latency interval. We would also expect a less than 1 to 2% risk of permanent neurologic morbidity related to the radiosurgery treatment. Before discussing the specifics of this case, it would be useful to review how our institution, the Massachusetts General Hospital, carries out SRS using a proton beam. As described previously, patients first undergo insertion of three 1/16-inch surgical-grade stainless steel fiducial markers into the surface of the skull under local anesthesia.45 During the same visit, custom dental fixation is made for a modified, relocatable Gill–Thomas–Cosman frame, and computed tomography (CT) scans are obtained and merged with previous images from MRI, CT angiography, or both, for radiosurgical planning for patients with an AVM. The target and nidus volumes are measured on the merged images, and custom-built brass apertures and range compensators are constructed. At the time of treatment, the patient wears the Gill–Thomas–Cosman frame and is placed into a calibrated robotic chair designed to move the patient and target into position for the immobile proton beam, which has the appropriate custom apertures and compensators for each convergent beam delivery. To calibrate this system, plain skull films are used to align the fiducial markers and match the preoperative imaging exactly (Fig. 17.2). For proton-beam SRS, treatments are done in one to two fractions with energy-degraded 160-MeV or 230-MeV beams at approximately 16 Gy (relative biological effectiveness) prescribed to a 90% isodose (Fig. 17.3). For further history and details, see Bussière and colleagues46 and Grusell and associates.47 Although the details of the actual procedure for proton-beam SRS vary, the basic concepts of preoperative image planning and stereotactic localization and calibration at the time of treatment remain the same across institutions. Although we are emphasizing proton-beam radiotherapy of AVMs in this discussion, it should be noted that much research on radiosurgery for AVMs has also been done with photons or other particles such as light ions. Hence, the studies cited here reflect a combined experience of SRS using protons, photons, or both. From an historical perspective, however, it is interesting to note that AVMs were one of the first neurosurgical conditions treated with proton-beam radiosurgery.48–50 At present, there is some debate regarding the necessity of treating AVMs, particularly to prevent the morbidity and mortality associated with them, including seizures, hemorrhage, and neurologic deficits.51 A thorough review of the natural history of AVMs is beyond the scope of this discussion, but current data suggest that previously unruptured AVMs have an annual hemorrhage rate ranging from 0.9 to 8%, according to one study,3 with similar findings from other research.2,52–55 Furthermore, several factors increase the baseline risk of hemorrhage, including a deep location, deep venous drainage, associated aneurysms, and previous hemorrhage.2,27,30,54,55 Although current research suggests that this patient’s AVM has a lower annual risk of hemorrhage given her age, the AVM location, and its superficial drainage, we would still recommend treating this lesion, particularly because the patient is symptomatic with seizures and has a significant cumulative lifetime risk of hemorrhage.32 Fig. 17.2a,b Plain skull films with fiducials marked as RA (right anterior), LA (left anterior), and LL (left lateral) in a patient with a midbrain AVM (circled). The patient is moved into position in a calibrated robotic chair to align the fiducial markers on these films with the predetermined plan. (a) Lateral view. (b) Oblique anterior view. (Courtesy of Marc Bussière.) The options for treatment include microsurgical obliteration, endovascular occlusion, and radiosurgery. Because the role of endovascular therapy as a primary treatment modality versus adjunctive therapy to radiosurgery and microsurgery is still being studied and the data are sparse, we shall defer further discussion at this time.56 In terms of surgery, several factors must be considered before offering surgical treatment. First and foremost, the goal of surgery is to improve the patient’s outcome, either by preventing future complications such as hemorrhage, seizure, and neurologic deficits, or by addressing current clinical symptoms. One study in children showed a 4% recurrence rate of AVMs after surgical obliteration.57 The consensus based on available data suggests that, in the proper hands of an experienced surgeon, obliteration rates can be very high, particularly for “low-risk” AVMs.53,58 In terms of seizure outcomes, our work at the Massachusetts General Hospital has shown surgery to be effective in obtaining Engel class I outcomes, although complete obliteration was more important than any specific treatment modality in predicting seizure outcome.20 Fig. 17.3a–d Axial (a), coronal (b), and sagittal (c) computed tomography (CT) images in a patient with a small right parietal AVM similar to that in the chapter’s presented case. This patient was treated with 15 Gy (relative biological effectiveness) normalized to an 87% isodose line. (d) The legend for isodose values. (Courtesy of Marc Bussière.) For neurologic outcomes, however, the situation is more challenging because surgery can address baseline neurologic deficits but also has a significant risk of causing neurologic deficits. Therefore, any discussion of efficacy and outcomes necessitates a discussion of surgical risk. In 1986, Spetzler and Martin6 published a landmark paper that risk-stratified AVMs according to size, location, and venous drainage. A Chinese group led by Shi and Chen also published a similar AVM grading schema in the same journal, but Spetzler and Martin’s scale has withstood the test of time as the basic method of communicating surgical risk associated with AVMs.59,60 The current patient’s AVM would be considered Spetzler–Martin grade II (SM-II) given its size of 1.5 cm, superficial venous drainage, and adjacency to eloquent sensorimotor cortex. According to their original paper, this ranking would confer a 95% chance of no deficit after surgical resection, with the remaining 5% for a minor deficit.6 In addition to the SM grade, factors that increase the surgical risk include the patient’s age, a diffuse nidus morphology, and a deep perforating arterial supply.7,61,62 Since the original paper by Spetzler and Martin, further studies have emphasized that surgical risk from retrospective analysis of single-surgeon/single-institution case series might reflect a selection bias.63 In fact, the surgical risk of 5% quoted for SM-II lesions might actually be as high as 9.5% in one study, based on the modified Rankin Scale as a more sensitive measure of outcome.63 In one series by Hartmann and associates,64 surgery for SM-II AVMs resulted in 36% of patients suffering from any type of neurologic deficit postoperatively. A series by Pik and Morgan12 reflects excellent outcomes for patients with low-grade AVMs but also suffers from an acknowledged selection bias. Lawton and his group61 have developed a supplementary scale to the Spetzler–Martin schema, which incorporates a ruptured presentation, the patient’s age, and diffuseness of the nidus. Based on the Lawton scale, the surgical risk for our patient, given her age of 40 years, the lesion’s unruptured presentation, and a presumed nondiffuse nidus, would be a 9.1% worse outcome as measured with the modified Rankin Scale score.61 Risks of surgery are significant, even with low-grade lesions in eloquent areas, and the approach can require additional surgical techniques such as language and motor mapping and stereotaxy.65,66 The purpose of this discussion is not to show that surgical obliteration is the “wrong” option, but rather to emphasize why radiosurgery is an appropriate first step for treating this patient. Radiosurgery can offer high rates of obliteration with low morbidity, and the immediate procedural risk and cost of microsurgery are much higher than radiosurgery. Those arguing against radiosurgery point to several complications: failure to obliterate lesions, hemorrhage during the latency period, and neurologic deficits from the effects of radiation. Although these risks are real, an appropriate understanding of how to choose patients for radiosurgery is critical and is discussed below. To frame the efficacy of radiosurgery as well as the risks, it is useful to review a new grading scale for radiosurgery developed by Pollock and Flickinger67,68 to emphasize the important factors that affect obliteration rates as well as neurologic deficits after radiosurgery. In their modified and updated scale, an AVM score is assigned according to the following equation: AVM Score = (0.1) (volume, mL) + (0.02) (age, years) + (0.5) (location: hemispheric, corpus callosum, cerebellar = 0; basal ganglia, thalamus, brainstem = 1) With a mean follow-up of 70 months (range 3 to 200 months), patients with AVM scores of less than 1 had an 89% obliteration rate without new deficits. For those with a score of 1 to 1.5, the rate was similar (70%).66 Higher scores, as expected, had lower obliteration rates. According to one study, factors that are associated with better outcomes (obliteration and limitation of any new neurologic deficit) include a smaller AVM volume, several draining veins, a younger age, and the location in the hemisphere.69 In the same study, AVMs smaller than 4 cm3 had a nidus obliteration rate of 83%.69 Obliteration rates ranging from 60 to 80% have been reported in similar studies, particularly for smaller AVMs (Table 17.3).16,70–83 Other groups have postulated relatively straightforward radiosurgery grading scales, such as that by Milker-Zabel and associates.84 Their research independently shows that obliteration is related to the patient’s age at treatment, the nidus volume, the maximum diameter, and the applied dose. Andrade-Souza and colleagues85 randomly selected patients from their experience with linear accelerator radiosurgery for AVMs and found that, of those with a Pollock–Flickinger AVM score of less than 1, 91.7% had excellent outcomes, which were defined as complete obliteration and no neurologic deficit. The Pollock–Flickinger score has been further validated in other studies as well.14,84–89 The AVM score developed by Pollock and Flickinger is a useful new tool to select patients and at present appears to be the new standard in discussing AVM radiosurgery. In terms of neurologic and functional outcomes, Pollock and Brown90 have shown that the modified Rankin Scale is an appropriate and sensitive measure of outcome after radiosurgery for AVMs. The modified Rankin Scale score has also been used as the outcome measure for microsurgery, making it an excellent standard for comparing outcomes among different modalities of treatment for AVMs, although further adoption of this measure is necessary.61–63 Outcomes have also been measured in terms of obliteration and neurologic deficit, with an excellent outcome defined as complete obliteration and no neurologic deficit.68 In one study, 11% of patients reported new or worsened neurologic symptoms,83 but in a similar study 95% of patients remained neurologically stable or improved.91 In a series examining radiosurgery on a majority of patients with AVMs who had undergone previous treatment, 88% did not have neurologic decline after radiosurgery, but there was a significant association between the size of the AVM and deficits after radiosurgery.89 Neurologic deficit after radiosurgery appears to be most related to the AVM volume and remains low across studies, particularly in subgroup analyses of smaller volume AVMs, a low Pollock–Flickinger AVM score, or both.14,53,68,80,87 In terms of seizure outcome specifically, Yang and associates92 have shown improvement after radiosurgery, particularly in those patients with documented obliteration of the AVM, a fact that is relevant to this patient and her presenting symptoms of seizure. Outside of neurologic complications, radiation itself can have an adverse effect on brain tissue, even without clinical manifestations. This effect can be seen as changes on MRI. Adverse radiation effects occur from occlusive hyperemia93 or radiation damage itself to adjacent structures. Ganz and associates94 showed that, for AVMs, there was a significant relationship between target volume and adverse radiation effects as measured by signal change on T2-weighted MRI. Hayhurst and coworkers95 found that target volume greater than 4 cm3 and a lack of prior hemorrhage were predictors of adverse radiation effects in their series. In the series by Kano and colleagues16 examining SM-I and SM-II AVMs, 2.3% of patients developed temporary symptomatic adverse radiation effects, with 1% developing permanent delayed cysts. After repeated radiosurgery, 4.9% of patients in the study of variable AVMs by Stahl and associates96 experienced adverse radiation effects. Our own group showed that, with proton-beam SRS specifically, the treatment dose and volumes were significant risk factors for radiation injury.97 Although the risk of radiation injury is not insignificant, it is low, particularly for small, conformal radiation fields.76,79,82 Should injury occur, however, surgical resection of any residual AVM and radiation injury can improve the clinical outcome.19 One of the major concerns and risks is hemorrhage during the latency period after radiosurgery but before complete obliteration of the lesion. Radiosurgery obliterates AVMs through progressive endothelial proliferation and occlusion of the AVM nidus over the course of years.98–100 In a study by Parkhutik and colleagues,98 AVMs that were not hemorrhagic at the time of presentation had a hemorrhage rate of 1.4% annually for the first 3 years followed by a 0.3% annual hemorrhage rate thereafter. The mean obliteration time was 37 months, which is consistent with a higher hemorrhage rate for the first 3 years when the majority of AVMs have not been completely obliterated. In their study looking only at SM-I and SM-II AVMs, Kano and coauthors16 reported a 3.7% annual hemorrhage rate the first year after radiosurgery and a 0.3% annual hemorrhage rate for postoperative years 1 through 5. As expected, factors that lead to higher baseline AVM hemorrhage rates, such as an associated aneurysm, were found by these authors to lead to higher post-radiosurgery hemorrhage rates.16 Similar rates were found by others.80,83,101,102 Turning to this chapter’s case presentation and the treatment decision, there are several factors to consider. Based on modern risk assessment, the patient’s surgical risk is as high as 9% for decline in the modified Rankin Scale score, a sen sitive outcome measure. Although surgery would likely obliterate the lesion immediately and decrease her seizures, it would also likely leave a deficit. If we calculate the modified radiosurgery AVM score (the Pollock–Flickinger score) for the patient, however, we find that it is 0.98 if we assume the volume of the AVM to be approximated to that of a sphere with a 1.5-cm diameter. Her modified AVM score would be less than 1 and she would have a 90% chance of obliteration with no risk of decline in the modified Rankin Scale score.68 Finally, if the AVM is not obliterated after the initial attempt at radiosurgery, it is safe to attempt a repeated treatment. Kano and associates16 showed that repeated radiosurgery is effective, particularly for patients with smaller residual nidus volumes and no previous hemorrhage. Other studies have also shown that repeated radiosurgery is safe and effective with limited complications.14,96,103 Some data also suggest that resection after radiosurgery is safe.104 Any discussion of radiosurgery for an AVM must mention some caveats. First, there is significant variability among studies, not only in the type of radiation applied, but also in how obliteration and neurologic outcomes are determined. Because AVMs are not as common as other neurosurgical conditions, the published experience from a single institution often spans more than a decade, during which time significant advances have occurred in the delivery of radiosurgery and in the decision-making process. When comparing studies or reviewing the literature, one must account for these inherent limitations. One of the most variable factors in radiosurgery is the applied dose, which is confounded by the types of radiation delivered (photons versus Bragg-peak particles including protons and light ions). A full discussion of dose is beyond the scope of this chapter, but it is important to realize that obliteration of AVMs after radiosurgery appears to relate to the radiation dose, particularly the marginal dose, and AVM volume, which are affected by the method of administering the radiation.82–84,86,89,94,101,103,105 Here, particle radiosurgery can take advantage of the steep dose drop-off of the Bragg peak to give more conformal and higher target volume doses with fewer side effects from spillover into adjacent sites.77,106 In addition, there is ongoing work on the role of fractionated radiotherapy versus radiosurgery for treating patients with AVMs.45,107 We strongly believe that the patient presented here would benefit from radiosurgery because it is safe and effective with a low chance of permanent neurologic deficit or radiation injury. Furthermore, the natural history of her lesion, based on current knowledge, does not put her at significant risk for hemorrhage during the latency period after radiosurgery. Her seizure symptoms should improve and ultimately, if radiosurgery fails to obliterate the lesion, microsurgery is a viable option without the patient having undertaken its risk upfront. The debate among microsurgery, radiosurgery, and, increasingly, endovascular treatments for both small and large AVMs will only grow as our strategies become more advanced. With regard specifically to radiosurgery, improved protocols for radiation delivery as well as more standardized outcome measures and grading scales will improve the generalization of data. The treatment of patients with an AVM, however, is still limited by its relatively low prevalence, the lack of randomized data, and the variability of factors associated with AVMs. Although our understanding of the natural history of cerebral AVMs continues to evolve, high annual risks of hemorrhage, ranging from 2 to 4% per year, have been documented in numerous series.1,22,30,31,52,54,55 In general, most patients warrant treatment for this reason. The threshold to intervene is lower in younger patients and in those with previously ruptured AVMs because these groups experience an increased cumulative lifetime risk of AVM hemorrhage.1–4,32 Furthermore, patients with unruptured AVMs that cause seizures, focal neurologic deficits, or both, are more likely to be offered treatment to improve or control the offending symptoms, while also reducing the risk of AVM hemorrhage. Unruptured AVMs may carry a lower hemorrhagic risk than ruptured ones.1,3 Thus, the benefit of intervention for unruptured AVMs is an area of recent controversy. Stapf and colleagues3 reported natural history data (mean follow-up of 829 days) obtained from a prospective database of 622 consecutive AVM patients evaluated at their center, the ARUBA study51 sponsor site; they identified multiple risk factors predictive of hemorrhage, including the patient’s increased age, a deep location, deep venous drainage, and a hemorrhagic presentation. In those patients with superficial, unruptured AVMs without deep venous drainage, similar to the patient described in this chapter, the hemorrhage rate over the study period was only 0.9% per year. Based in part on these data,108 the ARUBA study,51 which itself has raised controversy because of numerous concerns over its design,109,110 was initiated (and is ongoing) as a randomized trial comparing observation versus intervention (surgery, radiosurgery, embolization, or all) for patients with unruptured AVMs. However, as pointed out by Cockroft and associates,110 “it appears unlikely that any widely generalizable information will be obtained” from the ARUBA study because of its broad enrollment criteria, heterogeneous clinician and center experience, the variability in treatment modalities that are included, the inconsistent equipoise and associated selection bias, and an inadequate length of follow-up, among other factors. Because of predictably slow patient enrollment, the trial’s initial 30-month recruitment period was extended to 60 months, and the initial target sample size of 800 patients was reduced to 400 patients.51 Most unruptured AVMs should be considered for treatment, particularly in younger patients who would otherwise be exposed to the substantial risk of AVM hemorrhage over several years without treatment. For example, in a 40-year-old woman with an unruptured, superficially located AVM without deep venous drainage, who is expected to live another 40 years, if we assume that (1) the risk of AVM hemorrhage stays constant at 0.9% per year and (2) the hemorrhagic risk for any given year behaves independently of the other years, then by the multiplicative law of probability, her lifetime risk of AVM hemorrhage would be calculated as 30%.32 If instead we use the 4.3% per year hemorrhage risk in patients presenting with seizures, as reported by Ondra and colleagues,52 the calculated risk of hemorrhage over 40 years increases to 83%. Of course, hemorrhagic risk does not behave independently from year to year and would certainly increase if the AVM hemorrhaged; however, even our most conservative estimate suggests that the risk of AVM hemorrhage over a 40-year period is substantial. Ultimately, the decision to treat a given AVM must be individualized to account for numerous unique patient-specific and lesion-specific factors and cannot be reduced to any single grading scheme or statistical calculation. In the case presented, it is our position that the patient’s young age (implying both a longer cumulative life at risk and a better propensity to recover from potential treatment-related injury), her symptomatic presentation, a Spetzler–Martin grade of II, and a compact nidus all collude to indicate treatment. Let’s examine the various options. Irrespective of the treatment modality used, the AVM must be obliterated to reduce the risk of future hemorrhage; incomplete obliteration does not result in partial protection from,48,111 and may even increase the risk of,112 AVM hemorrhage. The more contentious issue is how best to achieve complete AVM obliteration with the lowest possible risk for a given patient. Should open surgery, radiosurgery, or embolization be offered? To cut, burn, or occlude? That is the question, among others, raised by the current case example and well addressed in the preceding sections. Complete, angiographically confirmed surgical AVM resection immediately and durably eliminates the risk of future hemorrhage in adults, and is generally considered the most effective treatment with the longest track record for this purpose.35,55 However, not all AVMs can be resected safely. In the past, some patients with large, complex, or small but deep-seated AVMs that today would be considered nonsurgical were operated on with significant morbidity, which was accepted as necessary to “defuse the bomb” in their heads. Fortunately, significant advances in the realms of radiosurgery and embolization have led to safer treatment pathways for some of these patients. Additionally, an increasingly balanced assessment of treatment risk against natural history has led to the recognition that certain AVMs should be followed or palliated rather than treated for cure, although some centers continue to push forward the frontier in this arena with multimodality treatment paradigms for even the most difficult lesions.8,11,12,35,36,55,64 Arteriovenous malformation surgical risk has been codified though incompletely addressed by numerous AVM grading schemes6,61,62,115–118; however, the Spetzler–Martin grade is the most widely used and takes into account AVM size, eloquent versus non-eloquent brain locations, and superficial versus deep venous drainage.6 In their original paper, Spetzler and Martin6 retrospectively applied the grading scheme to 100 consecutive patients who underwent complete AVM resection, and they were able to positively correlate the AVM grade with surgical morbidity. No patient with a low-grade AVM (I or II) experienced a major deficit. One of 21 patients with a grade II AVM (5%) experienced a minor deficit. In the follow-up prospective application of this grading scheme in 120 consecutive patients undergoing complete AVM resection, Hamilton and Spetzler36 correlated an increasing AVM grade with new transient and permanent neurologic deficits and reported that no patient with lesions of grade I or grade II experienced a permanent neurologic deficit. They also analyzed the individual components of the grading system and found that the AVM size and the pattern of venous drainage were each statistically significant predictors of the development of permanent neurologic deficits after surgery. An eloquent location alone was a statistically significant predictor of early transient but not permanent neurologic deficits. More recently, Lawton and associates61 introduced a supplementary AVM grading scale to account for three additional important features not addressed in the Spetzler–Martin scheme: the patient’s age, hemorrhagic versus nonhemorrhagic presentation, and a compact versus diffuse nidus. When applied to 300 consecutive surgically resected AVMs, this supplementary grading scale was a better predictor of the patient’s neurologic outcome after surgery than was the Spetzler–Martin grade, and the strongest predictive ability was achieved by combining both systems. As applied to the patient presented here, a 40-year-old woman with an unruptured AVM located in an eloquent area and with superficial venous drainage and a compact nidus measuring 1.5 cm, this lesion would be categorized as Spetzler–Martin grade II, supplemental grade III, with a combined grade (SM grade + supplemental grade) of V. Statistically, these scores predict a worsened neurologic outcome after surgery in 24.4%, 22.1%, and 21.1% of patients, respectively, according to the series by Lawton and colleagues.61 These results stand in contrast to those previously reported by Heros and associates35 and Hamilton and Spetzler,36 in which no permanent neurologic morbidity was reported in 98.7% and 100% of patients, respectively, undergoing resection of AVMs of SM grade I to II. One possible explanation for the discrepancy, among others, is that Table 5 in Lawton’s paper, in which the correlates of outcome are analyzed, is based on only 73 patients. Multiple series have suggested that small AVMs, even ones in eloquent areas, can be resected relatively safely in well-selected patients. For example, in a retrospective series of 100 consecutive surgically resected AVMs of less than 3 cm in size, Pik and Morgan12 reported neurologic worsening in two of 46 patients (4.3%) with AVMs in eloquent areas. In another retrospective series of 67 surgically resected AVMs of less than 3 cm in size, Sisti and associates8 reported permanent neurologic worsening in only one patient (1.5%); the proportion of AVMs in eloquent locations was not clearly specified but appears to have been at least 25%. In another retrospective series of 72 consecutive surgically resected AVMs, Pikus and coauthors11 reported that, in the 19 patients with AVMs of less than 3 cm in size, no new postoperative neurologic deficits occurred. Finally, Lawton and associates13 retrospectively analyzed technical and clinical results in 74 consecutively resected grade III AVMs and reported that, in the 35 patients with small lesions (< 3 cm) in eloquent areas with deep venous drainage, there were no new neurologic deficits after surgery, and there was one death secondary to fulminant liver failure of unknown cause, presumably unrelated to surgery. Small-sized grade III AVMs carried a lower overall surgical risk (2.9%) as compared with moderately sized (3–6 cm) lesions (7.1–14.8%). Arteriovenous malformation obliteration after radiosurgery occurs in a delayed fashion through radiation-induced endothelial cell proliferation, progressive vessel wall thickening, and eventual luminal closure.119–121 Although complete AVM obliteration rates reported after radiosurgery vary depending on the length of follow-up, the number of treatments attempted, the radiation dose used, and the imaging modality used (MRI versus cerebral angiography) to ascertain the final outcome, overall AVM obliteration rates ranging from 60 to 90% have been documented in numerous clinical series,16,69–71,77,80,122 and both a smaller AVM size and a higher radiation dose correspond with better obliteration rates after radiosurgery. A larger volume treated, a higher radiation dose, and repeated radiosurgery are also associated with higher rates of adverse effects, including cerebral edema and direct radiation-induced neurologic injury.16,94–96 On rare occasions, delayed rebleeding can occur even after complete angiographic obliteration is achieved.17 In a retrospective series of 217 grade I and II AVMs treated with radiosurgery as the primary therapy, Kano and colleagues16 reported obliteration rates of 58%, 87%, 90%, and 93% at 3, 4, 5, and 10 years, respectively. The median follow-up after radiosurgery was 64 months, the median time until total obliteration (as seen on MRI) was 30 months, and factors associated with a higher rate of total obliteration on univariate analysis included a smaller target volume, a smaller maximum diameter, and a higher margin dose. Thirteen hemorrhages (6%) occurred after radiosurgery, resulting in six deaths (2.8%) In another retrospective series of 300 consecutive radiosurgically treated AVMs, Liscák and associates80 reported a 74% obliteration rate occurring at a median of 25 months after initial radiosurgery and a total obliteration rate of 92% after repeated radiosurgery in patients with persistent AVMs 3 years after the initial treatment. Smaller AVM volumes and higher radiation doses were correlated with a higher chance of AVM obliteration. The cumulative risk of new permanent neurologic morbidity related to radiosurgery was 3.4%. Nineteen patients (6.3%) experienced AVM hemorrhage after radiosurgery, and three (1%) died as a consequence. The modified Pollock–Flickinger grading scale is a validated tool used to predict radiographic and neurologic outcome after AVM radiosurgery, and takes into account the patient’s age, the AVM volume, and the AVM location.68 In a series of 220 patients with radiosurgically treated AVMs, Pollock and Flickinger68 reported a 75% overall AVM obliteration rate and found that lower AVM scores, as calculated with their formula, correlated with higher obliteration rates without neurologic deficits. Twenty-three patients (9%) experienced hemorrhage after radiosurgery, resulting in nine permanent neurologic deficits (4%) and eight deaths (3%). As applied to the case described in this chapter, in a 40-year-old woman with a 1.5-cm parietal AVM, the modified Pollock–Flickinger score would be 0.98, which corresponds to an 89% chance of complete AVM obliteration without a new neurologic deficit after treatment.68 Embolization is typically used as a preoperative adjunctive therapy to facilitate surgical resection by devascularizing the nidus or through targeted occlusion of deep arterial feeders that are not easily accessible at surgery (or both). Although it is an important tool, embolization must be used judiciously, as it carries its own unique risks. For example, Taylor and colleagues123 reported a 6.5% permanent morbidity rate and a 1.2% mortality rate attributable to preoperative AVM embolization in 339 total procedures. We strongly believe that embolization of surgically accessible feeders is unnecessary and introduces the added risks of angiography and embolization, contrast agents, radiation exposure, and economic cost without significantly reducing the surgical risk. Additionally, embolization can be done to treat feeding artery aneurysms, typically in the setting of acute rupture, before later definitive therapy for the AVM itself. Less commonly, partial embolization is used to decrease the size of an AVM nidus to make it amenable to subsequent radiosurgical treatment; however, the utility of this technique is not well established. Indeed, prior embolization has been reported to be a negative predictor of outcome after AVM radiosurgery.69,124 Embolization as a primary curative therapy has generally been limited to small lesions that are typically supplied by a single vascular territory with arterial feeders that are accessible through a microcatheter. The same factors that make an angiographic cure possible after embolization, however, tend to also increase the likelihood of a low-risk surgical cure, but unlike the surgical track record, the durability of AVM obliteration after embolization alone remains to be established. On the other hand, highly selected patients with small inoperable lesions and favorable angioarchitecture for embolization or those who have accessible lesions but contraindications that preclude surgery can be considered for embolization; however, such patients are typically offered radiosurgery, which is the tried-and-true option. Nonetheless, embolization offers the possibility of an immediate angiographic cure that, if durable, constitutes an obvious advantage over radiosurgery, which typically requires 2 to 5 years to effect obliteration, with no protection from hemorrhage during the latency period. Onyx (ev3 Neurovascular, Irvine, CA) is now the liquid embolic agent of choice for AVM embolization. Compared with n-butyl cyanoacrylate, which was formerly the more widely used agent, Onyx carries less risk of microcatheter retention and can be injected slowly, in a more controlled fashion, to achieve deeper, diffuse AVM nidal penetration. As familiarity and experience with Onyx continue to accrue, the range of AVMs treated for cure with embolization will likely continue to expand. Although initial reports documented early obliteration rates with Onyx embolization ranging from 20 to 54%,38,39,125,126 recent studies suggest that short-term curative embolization can be achieved in 94 to 96% of well-selected patients.127,128 However, these are indeed highly selected patients and the results are nowhere close to general applicability. Long-term outcomes after curative AVM embolization remain unknown. The effect of AVM treatment on seizure outcome in patients presenting with seizures depends on a variety of factors reviewed elsewhere.20 Previous studies have suggested that about half of AVM patients are rendered free of seizures after either open surgery35 or radiosurgery129; however, more recent series have documented rates approaching 80%.130,131 In a series of 130 AVM patients with preoperative seizures who underwent surgical resection, 96% had a modified Engel class I outcome, characterized by freedom from seizures (80%) or only one postoperative seizure (16%) at a mean follow-up of 20.7 months.130 In another series of 86 AVM patients with a history of seizures treated with radiosurgery, 76.7% were free of seizure at a mean follow-up of 89.8 months; 96.7% of patients in whom the AVM was obliterated remained free of seizure, whereas only 30.8% were free of seizures when the AVM was not completely obliterated.131 In general, surgical resection and radiosurgery of AVMs appear to be comparable with regard to seizure outcome, and the patient described here has a good chance of complete freedom from seizure with either type of treatment, although this result would take longer to achieve with radiosurgery. Although we recognize that many practitioners would elect to follow this patient conservatively because her AVM is unruptured and in a so-called eloquent area, we would recommend treatment. She is young, the cumulative risk of rupture over the course of her expected lifetime is substantial even by conservative estimates, she has a better potential to recover from postoperative sequelae, and her AVM is small, superficial, compact (as opposed to diffuse) and situated posterior to the sensory cortex. Not to belittle the use of “eloquence” as simplified and categorized by Spetzler and Martin, but it escapes no one that the concept has been oversimplified for the purpose of convenient categorization—hence its utility and limitations. All eloquent brain is not created equal. A small AVM in the sensory cortex can be treated with less risk than a small AVM in the brain stem or thalamus. On the other hand, we respect the fact that significant injury to the sensory cortex could also result in significant disability for the patient, including the disturbing alien hand syndrome. Radiosurgery is a reasonable option, which avoids the upfront risks of surgery with a high likelihood of complete AVM obliteration within 3 to 5 years. However, because there is no protection from hemorrhage during the latency period, because this continued risk of hemorrhage naturally carries more severe consequences when the AVM is situated within an eloquent location, and because there is the possibility of devastating, delayed, radiation-induced injury to the sensory cortex, a compelling argument can be made for surgical resection, if it can be done safely. To this end, we would obtain a preoperative functional MRI scan with diffusion tensor imaging and fiber tractography to guide our resection, accepting the small risk of injury to the posterior sensory cortex. Figure 17.4 illustrates these points in a similar patient we treated successfully through surgery, in whom the AVM directly abutted the anterior bank of the motor cortex. Figure 17.5 illustrates the potentially devastating, and not so rare, consequences of radiosurgery in AVMs in eloquent areas, which necessitated surgical resection in another patient. If radiosurgery were done up front in the sample case described here, with a 90% chance of complete obliteration in 3 to 5 years, what would be the next course of action for the 10% chance that obliteration was not achieved? Radiosurgery could be repeated, with an increased risk of adverse radiation effects (5%)96 and with a continued hemorrhagic risk during the second latency period. On the other hand, previous radiosurgery has been reported to facilitate AVM resection while reducing surgical morbidity.104 Both repeated radiosurgery and surgical resection are valid options in this scenario, and the same considerations apply. Managing an AVM requires an understanding of its natural history, which must be balanced against the risks of treatment before reaching a well-informed decision. Even when armed with this understanding, experts in the field may have divergent opinions, as this and the preceding chapters illustrate. Every AVM and every patient is unique, and no single grading scheme or statistical calculation can completely dictate the appropriate strategy for a given case. In fact, the final treatment decision, in this practical world of ours, very much depends on the available resources and experience of the surgeon and center at which the patient is seen. Although it goes without saying that a multidisciplinary approach is laudable, ultimately, experienced neurosurgeons must strive to know the indications, efficacy, and complications of all potential treatment modalities, including surgery, radiosurgery, and embolization, in their own hands, in their own center, to counsel patients appropriately, especially in the face of controversy. Multidisciplinary consultation works best when the learned opinions of the many contribute the necessary elements of experience that enrich the wisdom of the decision of the one and final decider. Such consultation, unfortunately, is misused all too often as a vehicle to abrogate individual responsibility and to diffuse, rather than consolidate, the sense of moral obligation to the patient. Treatment by committee works no better than having several people maneuver the steering wheel of one car. No single person will feel responsible for the crash. One leader and driver is indispensable. And, at the risk of preaching fiscal heresy and disregard for the “bottom line” of hospitals and physician practices, the ethical practitioner who recognizes that the best treatment may not be available at his or her own facility should refrain from resorting to the too commonly used strategy of prescribing “what is available at our center” rather than prescribing what is best for the patient at another center. In the final analysis, if the patient discussed here were a patient of the senior moderator at the University of Miami, she would undergo surgical resection.
Treatment of an Arteriovenous Malformation in Eloquent Areas
Microsurgery for Small Arteriovenous Malformations
Natural History
Microsurgery
Radiosurgery
Case Discussion
Conclusion
Gamma Knife Radiosurgery for Arteriovenous Malformations
Proton Beam Stereotactic Radiosurgery for Arteriovenous Malformations
Procedure
Natural History
Treatment Options and the Case for Microsurgery
Radiosurgery
Grading Scales
Outcomes and Adverse Radiation Effects
Hemorrhage
Decision Making
Caveats
Conclusion
Moderators
Treatment of an Arteriovenous Malformation in Eloquent Areas
To Treat or Not to Treat?
How to Treat?
Surgical Resection
Radiosurgery
Embolization
Seizure Outcome
Discussion
Conclusion
< div class='tao-gold-member'>
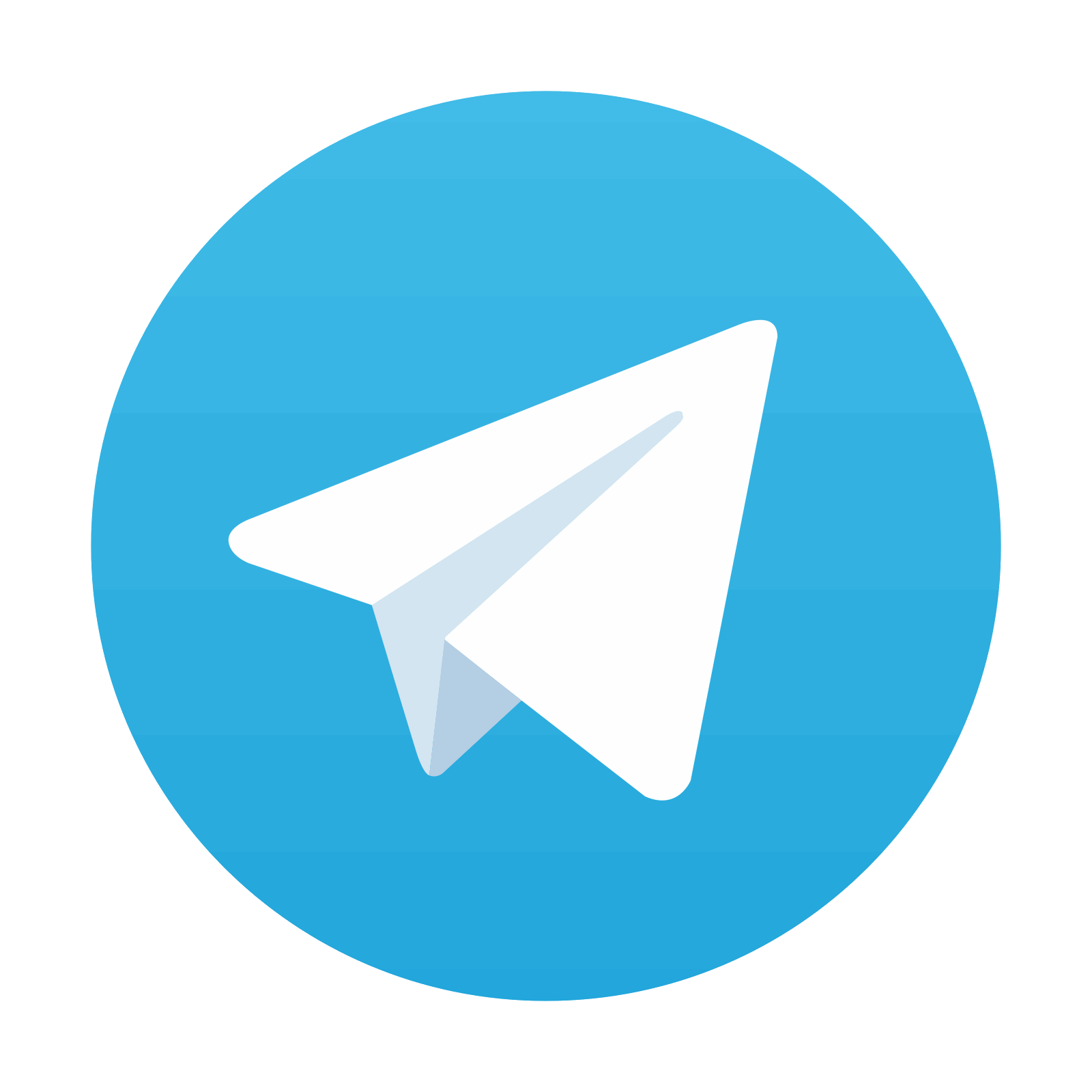