Fig. 6.1
Stationary mount used to photograph the specimens. Shown is a rib model on the grid used to obtain high-resolution photographs in six projections
A total of 6226 high-resolution photographs of ribs and vertebra of the 32 pediatric specimens were obtained. All available ribs and spines were photographed. Ribs were sorted right to left and proximal to distal, labeled as ribs 1 through 12, and were imaged from three orthogonal angles, with the digital camera mounted on separate. A tripod vertebra were arranged from C1 to L5 and photographed in six positions – anterior, posterior, right lateral, left lateral, inferior, and superior. Each photograph was calibrated from a fixed background grid, and quantitative measurements were taken. Scandium Image Analysis Software (Olympus, Soft Imaging Solutions) was used for all measurements, reported to 0.1 mm. The final data set has resulted to date in over 32,000 separate measurements.
There are limitations to using osteology collections in the study of human growth. These include a small sample size, assumption that all specimens are normal, selection bias inherent in using only available specimens (i.e., a bias to poor undernourished children) and cross-section data being used to infer longitudinal normal growth of both individuals and of populations. Other limitations include the following: specimen height and weight were not included in the measurement analysis and the actual chest volume could not be assessed due to inability to measure soft tissue structures. The method used for measurement was subjective, with the points of interest being chosen by the investigators. Test, re-test, inter- and intra-observer reliability was not performed; however, the variability of these lineal measurements in preliminary pilot testing was small.
6.2 Rib Growth
6.2.1 Background
During human evolution of Homo erectus, the rib cage transitioned from a triangular funnel shaped to a barrel shaped chest approximately 1.6 million years ago [6]. This is believed to be a result of environmental changes to a more rich and efficient diet that allowed the abdominal organs and waist to narrow. The resulting change in trunk contour is believed for the first time to allow efficient running, which likely provided further evolutionary advantages. There is little detailed information how the triangular-shaped neonatal thorax assumes the barrel shape of adulthood. With increasing age, the chest transitions from a circular to an elliptical cross-section shape with greater transverse width in the mid-thoracic spine at ribs 6 and 7 [7]. Individual ribs have very distinguishing features with the more proximal ribs being flatter and more curved than the straighter lower ribs (Fig. 6.2).
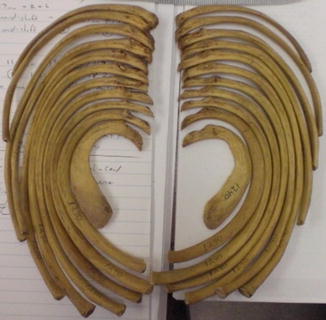
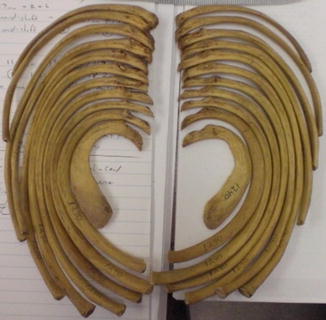
Fig. 6.2
Specimen from the Hamann-Todd Collection showing ribs 1–12. Rib 1 (inside in this photograph) is flatter and more curved than the lower ribs (outside ribs). The longest ribs are in the mid-thorax, creating the frontal plane barrel shaped thorax seen beyond infancy
The rib articulates with the thoracic vertebra at the superior lateral aspect of the vertebra and the tip of the transverse process. This provides both stability to the thorax and an axis of rotation for the rib for providing chest motion for respiration (Fig. 6.3). Dimeglio has provided contemporary information on how the chest grows in volume during childhood [1]. The neonate has approximately 6 % of eventual adult chest volume. Although the 10-year-old child is nearing adult height, by maturity, there has been a doubling of chest volume beyond age 10 years. The changing length and shape of the rib create a greater cross-section of the thorax, that when added to the increase in thoracic height, rib separation and costo-sternal growth contribute to overall thoracic volume. Whereas the growth of the long bones is well described, basic rib growth has not [8]. In the human femur, 70 % of the growth occurs distally (approximately 1 cm/year), with 30 % of growth occurring proximally. For the humerus, 80 % of linear growth is proximal and 20 %, distal. Rather than describing normal rib growth, the majority of published rib studies have described abnormalities of rib or thorax growth and asymmetry in children with scoliosis [9–14]. Rib deformities created in experimental animals have demonstrated that abnormal thoracic development is a common feature of scoliosis [15, 16].
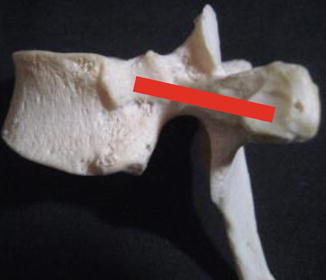
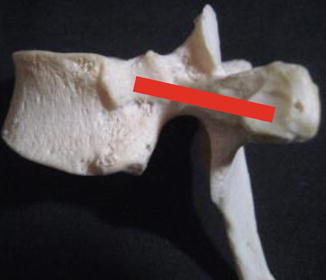
Fig. 6.3
Left lateral view of a mid-thoracic vertebra. The red line defines the axis of rib rotation. This axis is relatively more oriented in the coronal plane in the upper thoracic spine and more sagittal oriented in the lower thoracic spine
Although our study did not look at specific growth in an individual, by looking at a spectrum of growth over the entire period of childhood for a small population, these data can provide some insight into how the individual child’s ribs grow. Introducing standardized definitions of the dimensions of the rib has allowed us to describe normative data for how the rib appears to grow during childhood. The area described by the shape of the rib does not describe the total chest volume, which includes the anterior cartilage and sternum, spine, diaphragm, rib spacing and other soft tissues of the thorax. Sandoz et al., in a normative cross-section CT study, showed that the costal cartilage accounted for 45–60 % of rib length, which is a feature of thoracic volume that cannot be studied in an osteology collection [8]. Since we were most interested in the area enclosed by the rib shape, we defined two measurements that represented this space. The outer costal length (OCL) is the total curved length of the rib, and the base diameter (BD) is the linear distance connecting one end of the rib to the other (Fig. 6.4). These two measurements influence the shape and size of the chest and therefore are two of the key parameters in thoracic growth and development. Of the 32 pediatric specimens, 714 total ribs were present and intact for measurement. This allowed us to make inferences about the unique shape and growth pattern of pediatric ribs and determine how the growth of the rib contributes its part to the volume and shape of the developing pediatric thorax.
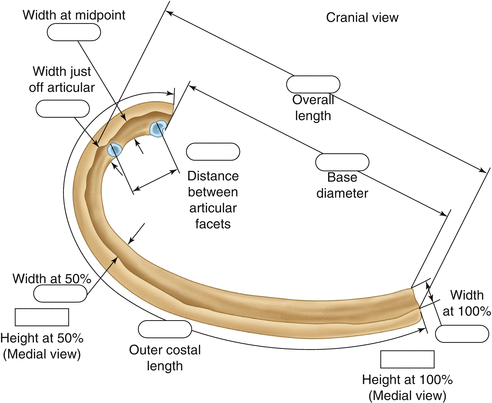
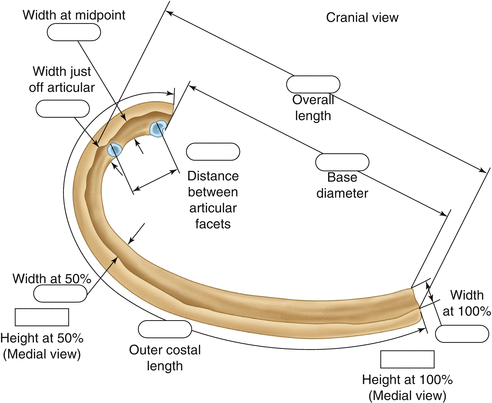
Fig. 6.4
Measurements of the rib outer costal length (OCL) and base diameter (BD) are illustrated
6.2.2 Linear Rib Growth
Each rib has its own linear growth rate, which more than tripled in length from birth to adulthood. The fastest growing ribs are the mid-thorax ribs, which may influence the triangular shape of the neonatal thorax to become the barrel shape of the older child and adult thorax [9]. There is coupled growth among the upper, middle and lower ribs. Thus, rib pairs number 1 and 12, 2 and 11, 3 and 10 and the middle ribs as a group are noted to have the projected area increase with increasing specimen age. The lower ribs where the diaphragm is attached are straighter than the more curved upper ribs. Table 6.1 shows the linear rates of rib growth by vertebral level. The middle ribs increase in length as well as projected area the greatest, similar to the growth rate of the distal femur (Fig. 6.5).
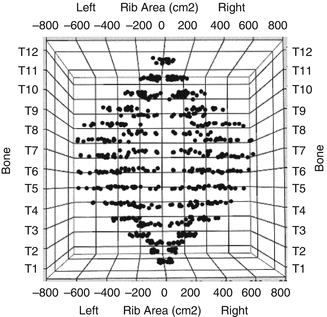
Table 6.1
Growth rate of ribs 1–12, aged 1 year to 18 years
Rib | Starting length (mm) | Growth rate (mm/year) | Correlation coefficient |
---|---|---|---|
1 | 56.7 | 4.1 | 0.761 |
2 | 86.9 | 7.5 | 0.823 |
3 | 99.5 | 9.4 | 0.869 |
4 | 105.0 | 10.3 | 0.823 |
5 | 112.0 | 11.0 | 0.864 |
6 | 114.2 | 10.7 | 0.854 |
7 | 113.9 | 10.6 | 0.821 |
8 | 109.6 | 10.5 | 0.885 |
9 | 106.4 | 9.4 | 0.842 |
10 | 94.5 | 8.1 | 0.823 |
11 | 69.9 | 6.1 | 0.760 |
12 | 39.0 | 4.0 | 0.575 |
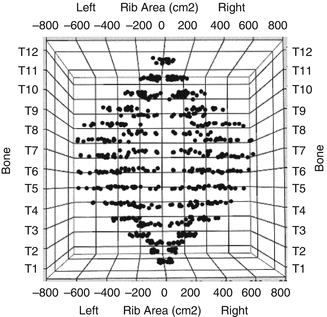
Fig. 6.5
The mid-thoracic ribs increase the greatest in projected area for all ages. This effect is especially notable in the older adolescent, where the barrel-shaped chest is apparent. There is symmetry in projected area between left and right ribs. Younger subjects (aged 1 and 3 years) appear near the center of the graph, while older subjects (aged 17–18 years) are at the far left and right
6.2.3 Coupled Symmetry of Growth
The two physical measurements taken from the ribs for this study (OCL and BD) showed that ribs in these children follow specific patterns of growth. Both the OCL and BD increase with age. An important finding was the linear and volumetric growth and concept of coupled symmetry (Fig. 6.6). The ribs of the upper and lower thorax are shaped very differently, yet they attain the same projected area through different means. The upper thorax contributes to area more by an increase in the OCL, while the lower thorax adds area by an increase in the BD. The lungs are broader at the diaphragm than at the proximal end, and the shape of the ribs helps determine this. The projected rib area greatly increases after age 10 years, reflecting the known doubling of thoracic growth after this age (Fig. 6.7).
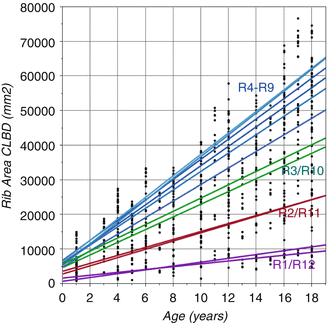
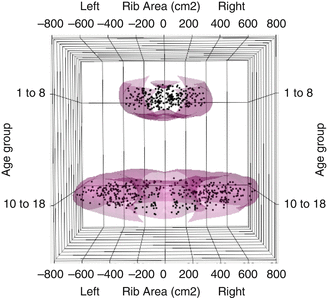
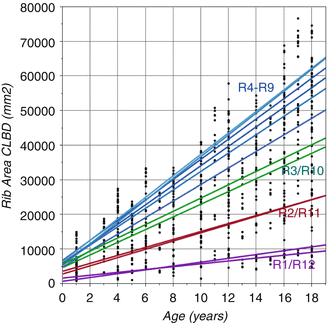
Fig. 6.6
Increasing projected rib area with increasing age. The middle ribs (R4–R9) are increasing the greatest with age. There is coupling of growth between the upper ribs and the lower ribs
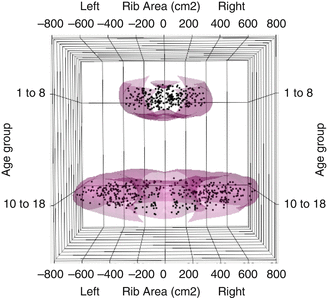
Fig. 6.7
Projected rib area by age group, 1–8 years and 10–18 years. There is a marked increase in projected area after age 10 years, which parallels the known doubling of thoracic volume that occurs after age 10 years
6.2.4 Logarithmic Spiral
We observed that the changing curvature of the rib resembles the well-described principle of growth of solid structures in nature, the logarithmic spiral (Fig. 6.8). This is a geometric principle that is seen throughout nature in animals with shells and is a common mechanism for how rigid organisms increase in volume without changing their basic shape. A logarithmic spiral pattern is also seen in nature in spider webs, low-pressure weather patterns and some interstellar structures such as the Whirlpool Galaxy [17]. Although often described as a golden spiral, these spirals in nature are more accurately logarithmic spirals. Their shape is sometimes approximated by the Fibonacci sequence of numbers, the golden rectangle or the golden ratio. The golden ratio is based on the number phi (1.618) and has a ratio of sides of 1.618 to 1.0. A sampling of over half of the available 714 ribs were evaluated for how closely they followed these spiral forms. The ribs deviated from the expected spiral with an average error of ~8 %. Most of the 1 cm/year of growth of the middle ribs seems to be creating volume by growth of the anterior sternal aspect of the chest, similar to how a nautilus grows. This amount of linear growth of the middle ribs is comparable to that of the distal femur, the fastest linear growing long bone in the human.
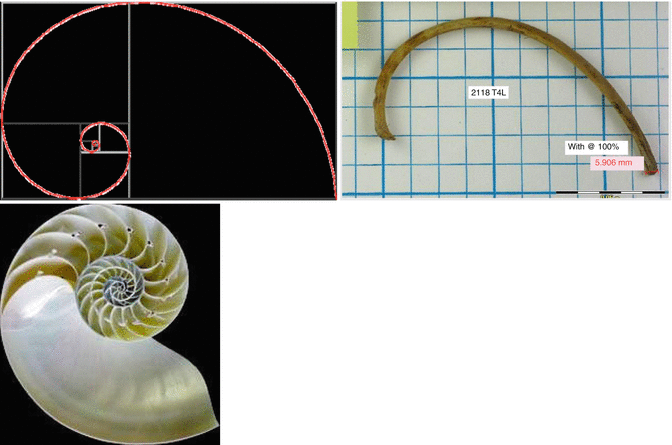
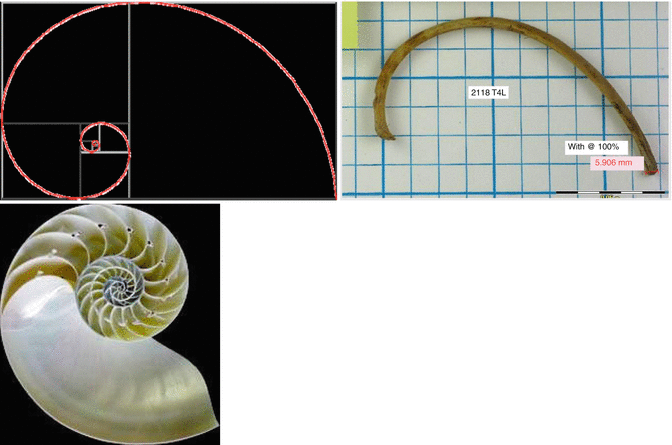
Fig. 6.8
The human rib appears to follow the elliptical spiral structure found in nature with the majority of its growth at the sternal end
Other rib measurements of interest are the rib height and width, which were included in our measurements. We determined that rib height reaches one-half of adult rib height by 1 year of age but still increases in height into adolescence. In contrast, rib thickness reaches close to adult size by about a year of age. This confirmed a CT study that we had previously performed on a normative pediatric population that showed an average rib height of 6.9 mm (one-half adult rib height) by 1 year of age and reaching three-fourths of the adult rib height by 3 years of age. Compared to the growth of the more distal body segments such as the femur, there appears to be early preferential growth of the thorax and ribs. This is particularly important since the upper ribs are frequently used for hook anchor sites. Ferguson et al. [18] showed that the greatest available width of the rib for implant attachment is just lateral to the transverse process: T2 width was 8.4 SD 1.2 mm; T3, 6.3 SD 1.0 mm; T4, 5.6 SD 1.1 mm; T5, 5.0 SD 0.7 mm.
6.3 Vertebra Growth
Initially, we used electronic calipers to obtain morphometric measurements of the vertebral bodies (Fig. 6.9). With time, it became apparent that high-resolution photographs could more quickly provide this information. Six photographic images were obtained for each specimen: anterior, posterior, right lateral left lateral, superior and inferior. Data were plotted for vertebral body width and height with age (Fig. 6.10). The data show linear growth of the vertebral bodies. However, the data are insufficient to determine if this is continual linear growth or is curvilinear growth with different velocities at different ages. By age 1 year, the vertebral width, but not height, is one-half the eventual adult size. Vertebral body width and height increases are greater with more distal levels, and growth continues into late adolescence (Fig. 6.11). Transverse process height and width dimensions were also obtained at levels T1, T4, T7, T10 and L3 for 16 representative specimens. By 1 year of age, these TP dimensions had attained one-half of their eventual adult dimensions, similar to rib height and vertebral body width.
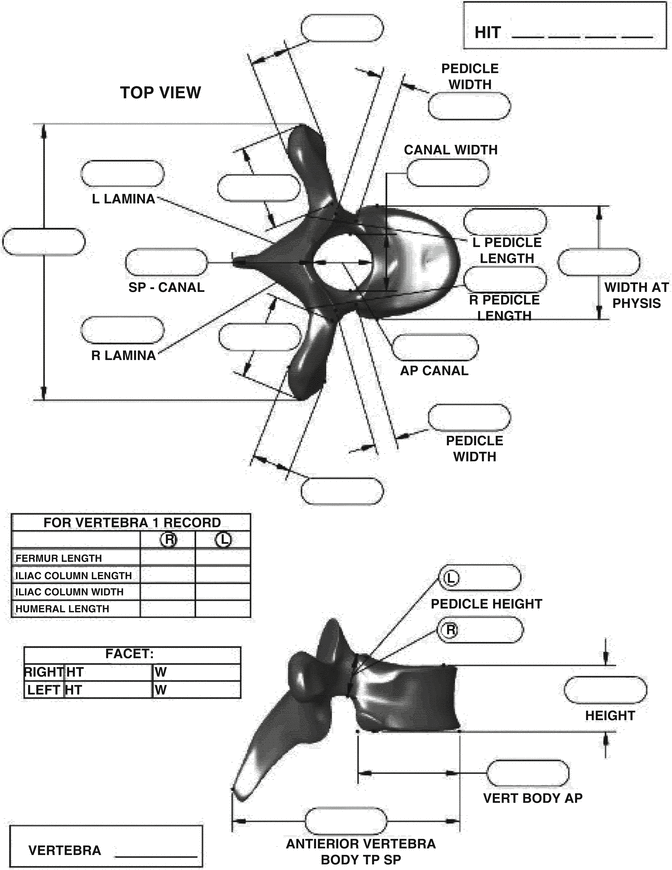
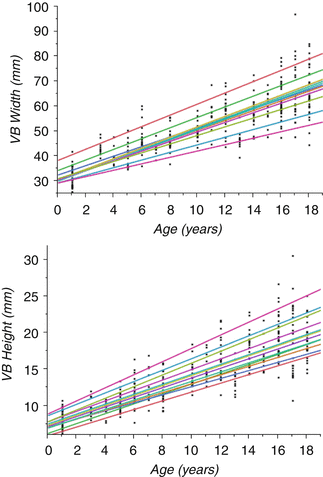
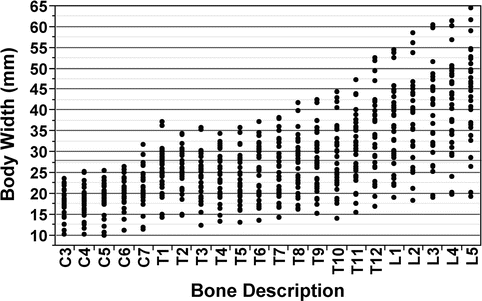
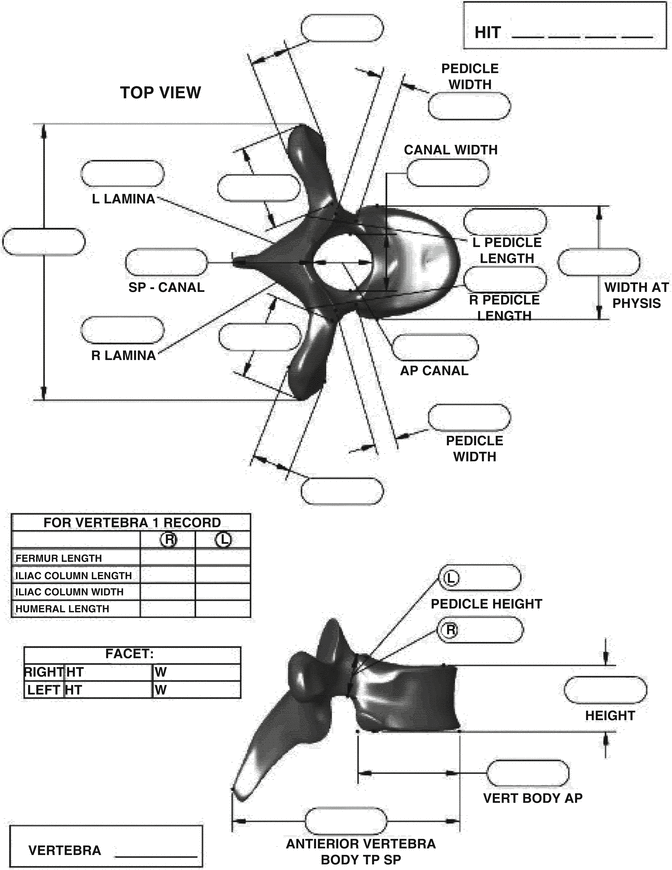
Fig. 6.9
Superior and right lateral views of vertebral body showing the measurements obtained by both calipers and photo imaging
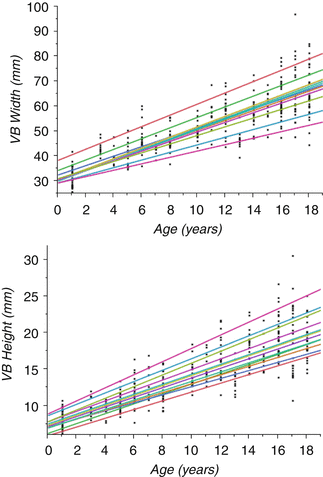
Fig. 6.10
Vertebral body width increases at 1.9–2.1 mm/year r 2 = 0.78–0.86. Vertebral body height increases at 0.55–0.90 mm/year, r 2 = 0.8–0.87. Each line in each figure represents the linear regression for T1–T12, with T12 the bottom line and T1 the top line
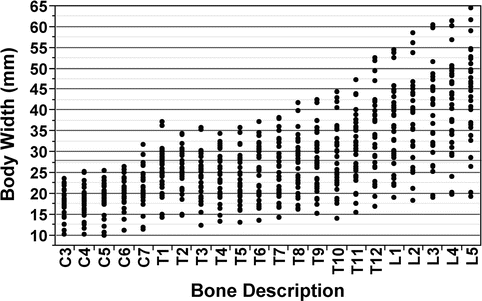
Fig. 6.11
Vertebra body width for each vertebral level. The spread for each vertebral level indicates increasing width with age from 1 to 18 years. C1 and C2 data are not included. Vertebral body increases in width with more distal segments. Younger subjects (aged 1 and 3 years) are at the bottom of the graph and older subjects (aged 17–18 years) are at the top
6.4 Spinal Canal Growth
6.4.1 Background
The spinal canal enlarges early in life through endosteal remodeling of the posterior elements and longitudinal growth of the pedicles through the neurocentral synchondrosis (NCS). Osteological studies have traditionally been used to describe normal vertebral canal dimensions and growth [19–22]. Most osteological studies show that the lumbar AP canal diameter reaches adult size very early at approximately 3–5 years of age, whereas transverse diameter continues to grow into adolescence [23]. Porter et al. [23] demonstrated that by 4 years of age, the mean inter-pedicular diameter was 87 % of adult size and that the vertebra canal area was completely mature. Dimeglio [3] has stated that the spinal canal has reached 95 % of its adult area by 5 years of age. However, there is evidence in osteological collections that inter-pedicular diameter increases until about age 10 years, suggesting that the canal area is also increasing [24]. Environmental factors and health may also contribute to growth inhibition and spinal stenosis for which catch up growth is difficult [22, 24]. Papp et al. [24] also showed that the proximal spine segments mature before the more distal lumbar segments.
Besides the canal becoming wider with growth, the pedicles also increase their transverse width with age. The increase in pedicle size occurs lateral to the spinal canal through remodeling (Fig. 6.12). The implication of this outer pedicle wall remodeling is that this allows the medial pedicle wall to become thicker and more resilient to inadvertent medial pedicle wall implant penetration and also provides for increased canal transverse diameter as the pedicles enlarge. Human and animal studies have shown that unilateral surgical closure of the neurocentral synchondrosis can cause asymmetric growth and scoliosis [25]. However, these procedures do not cause significant spinal canal stenosis, likely due to preserved posterior element appositional growth.
