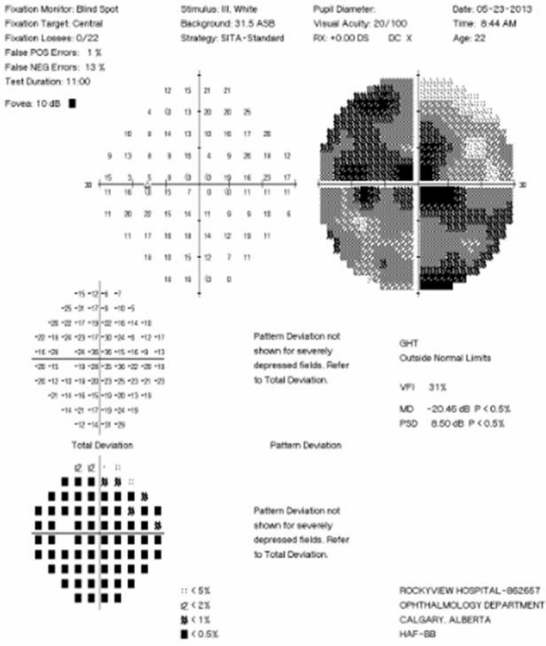
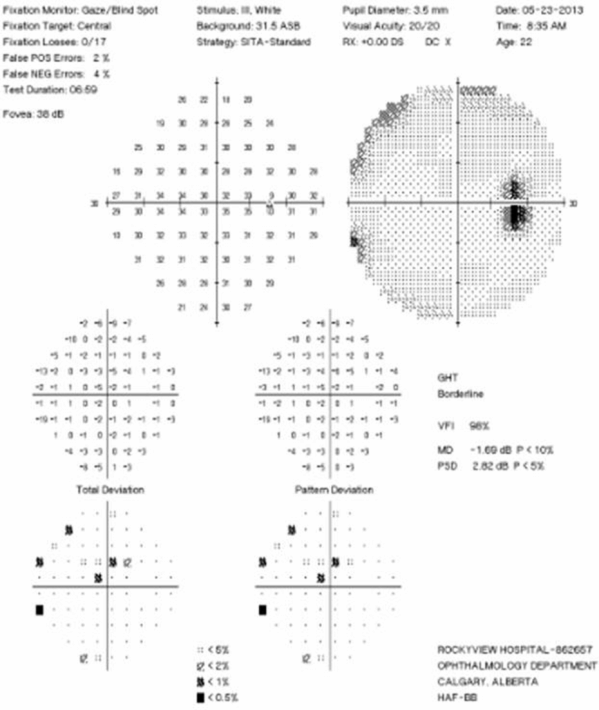
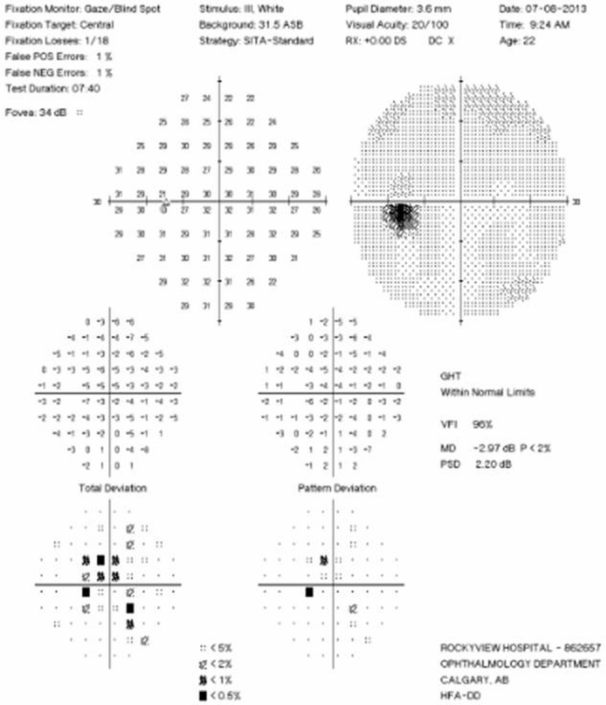
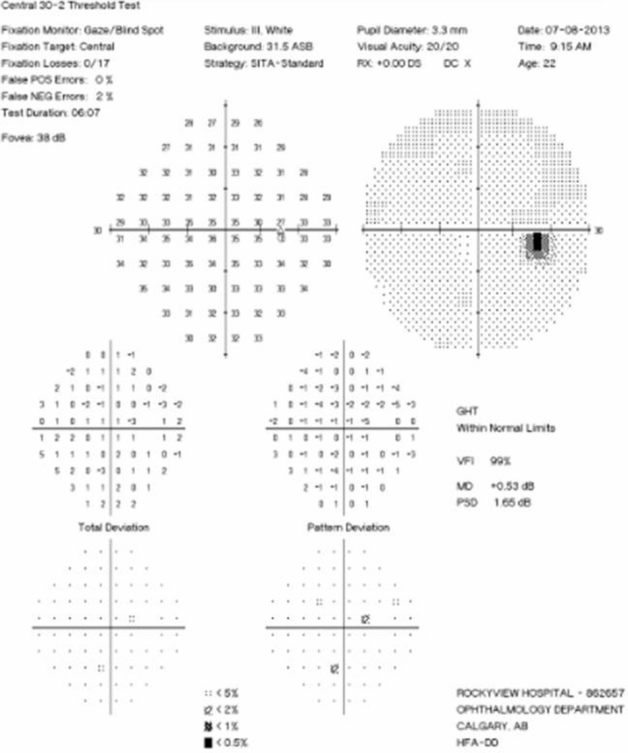
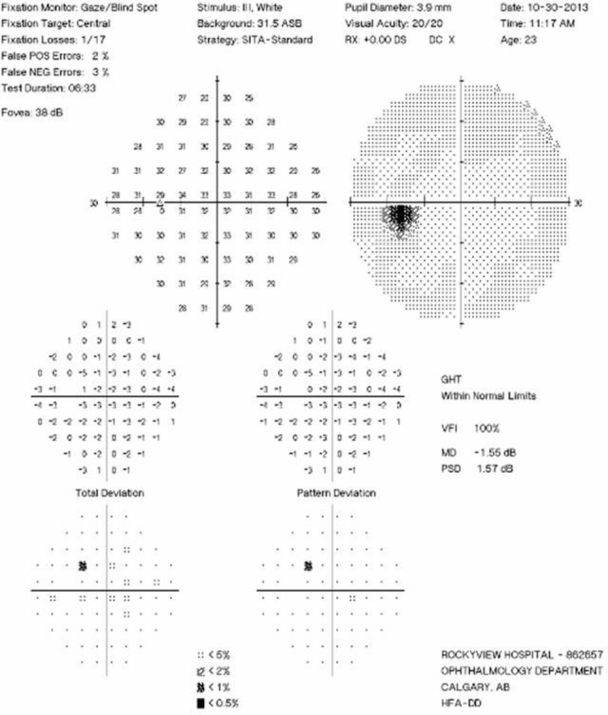
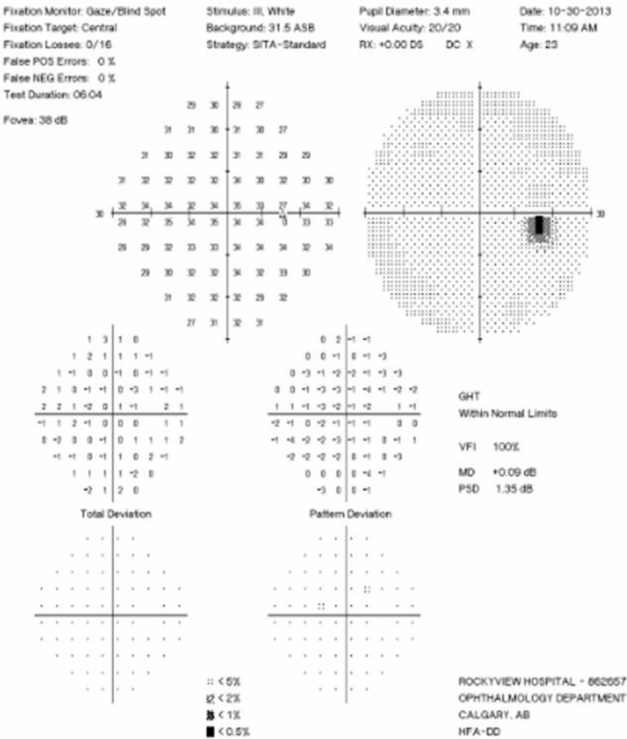
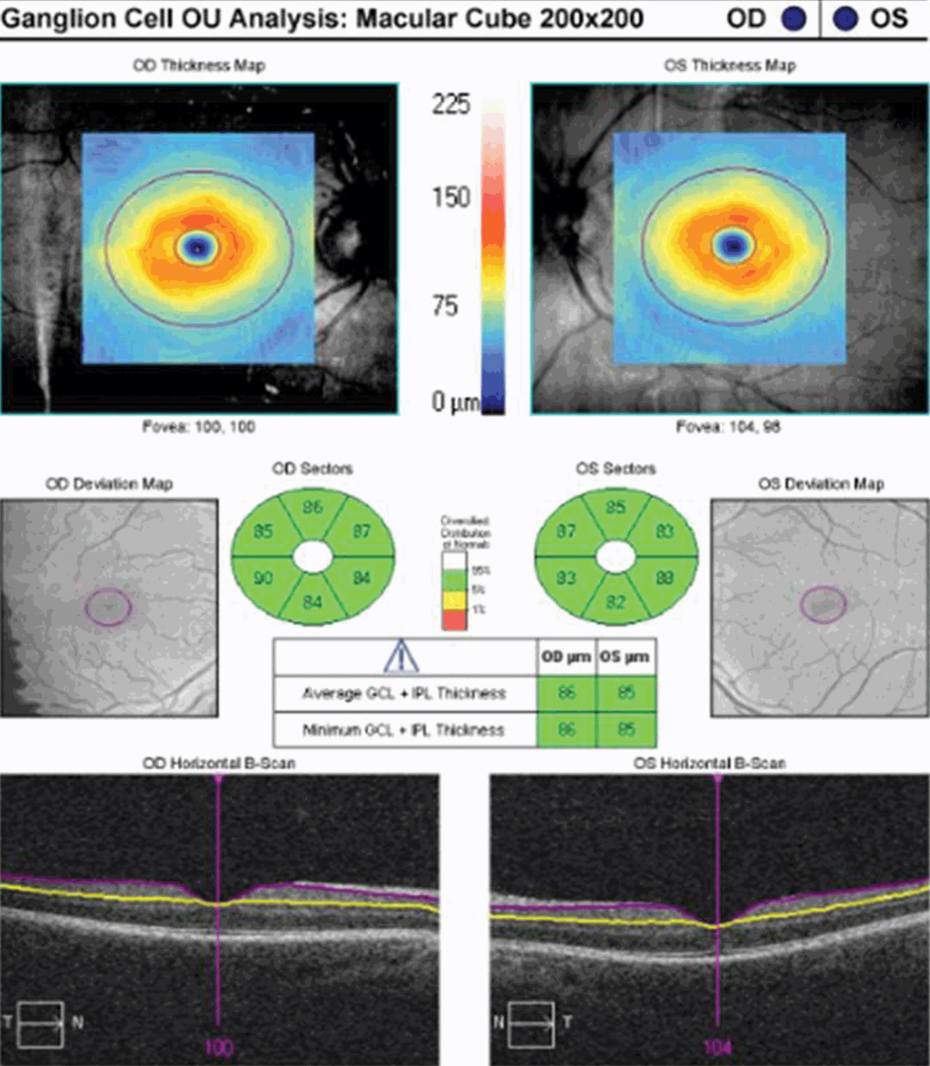
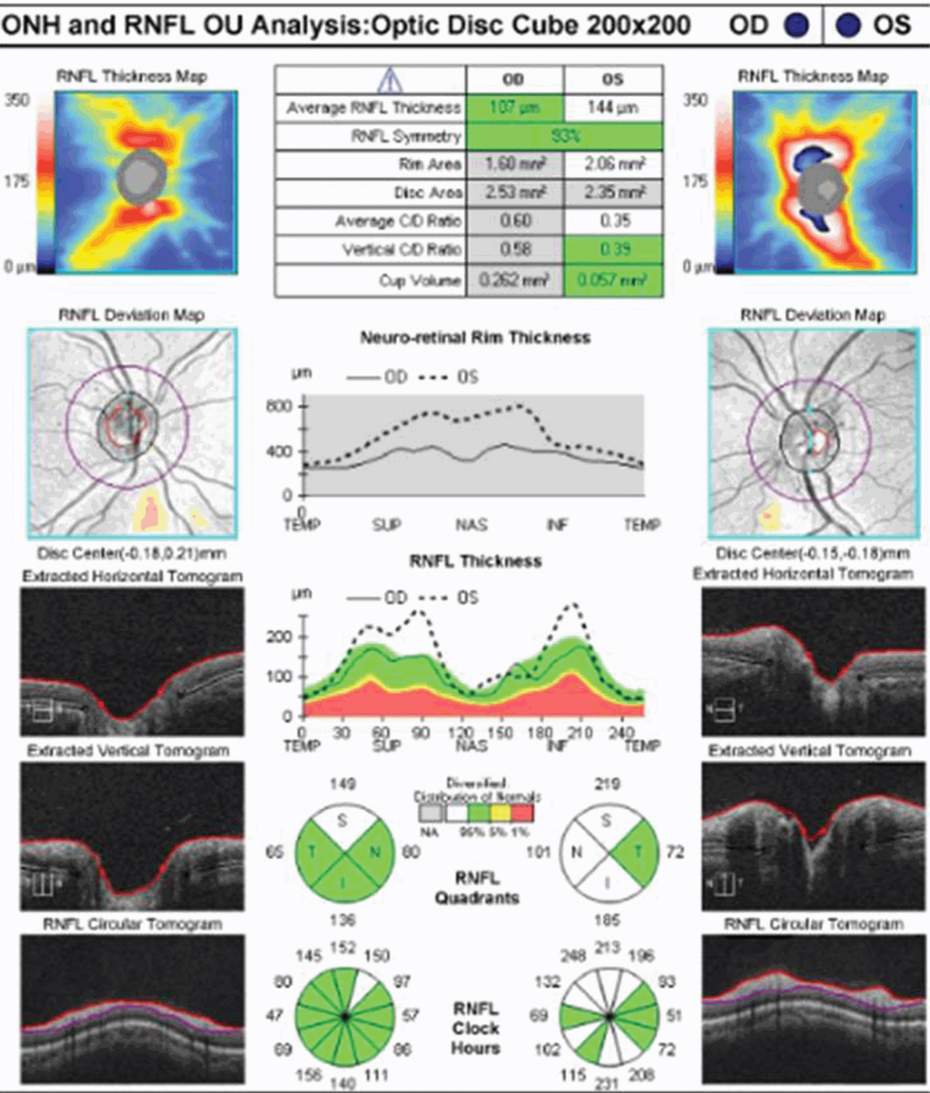
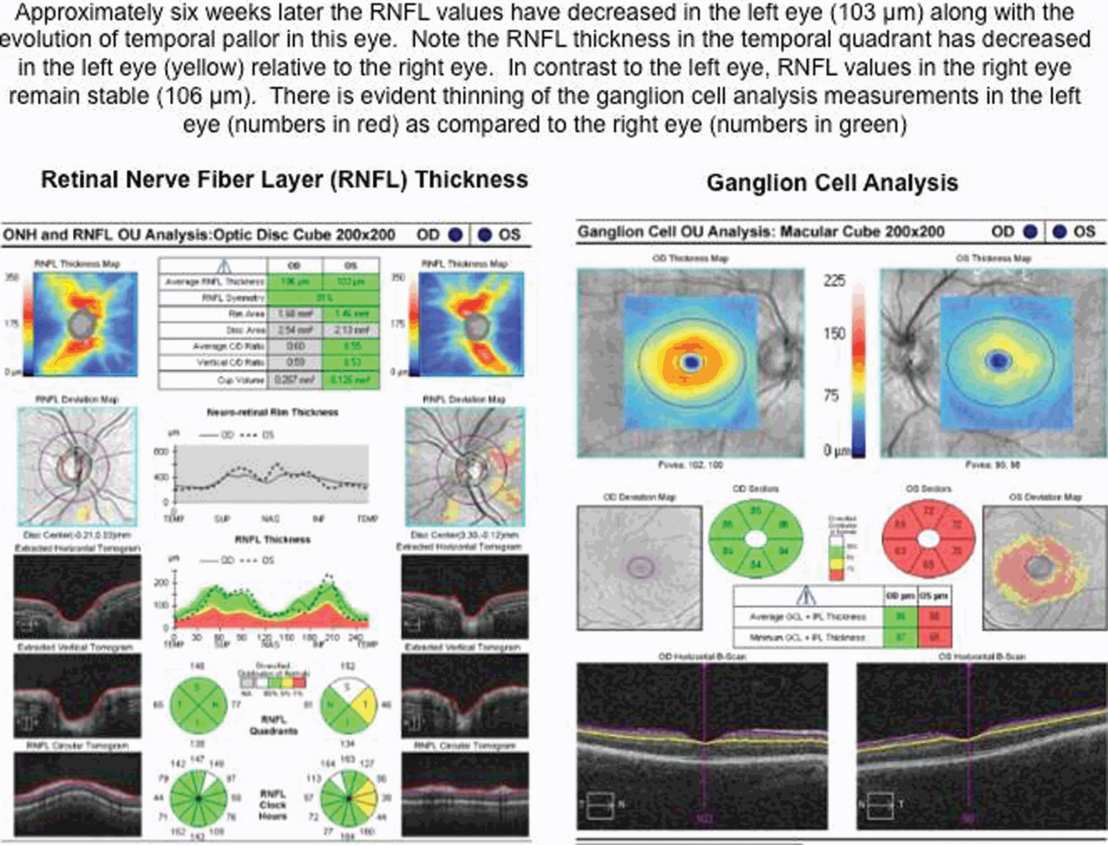
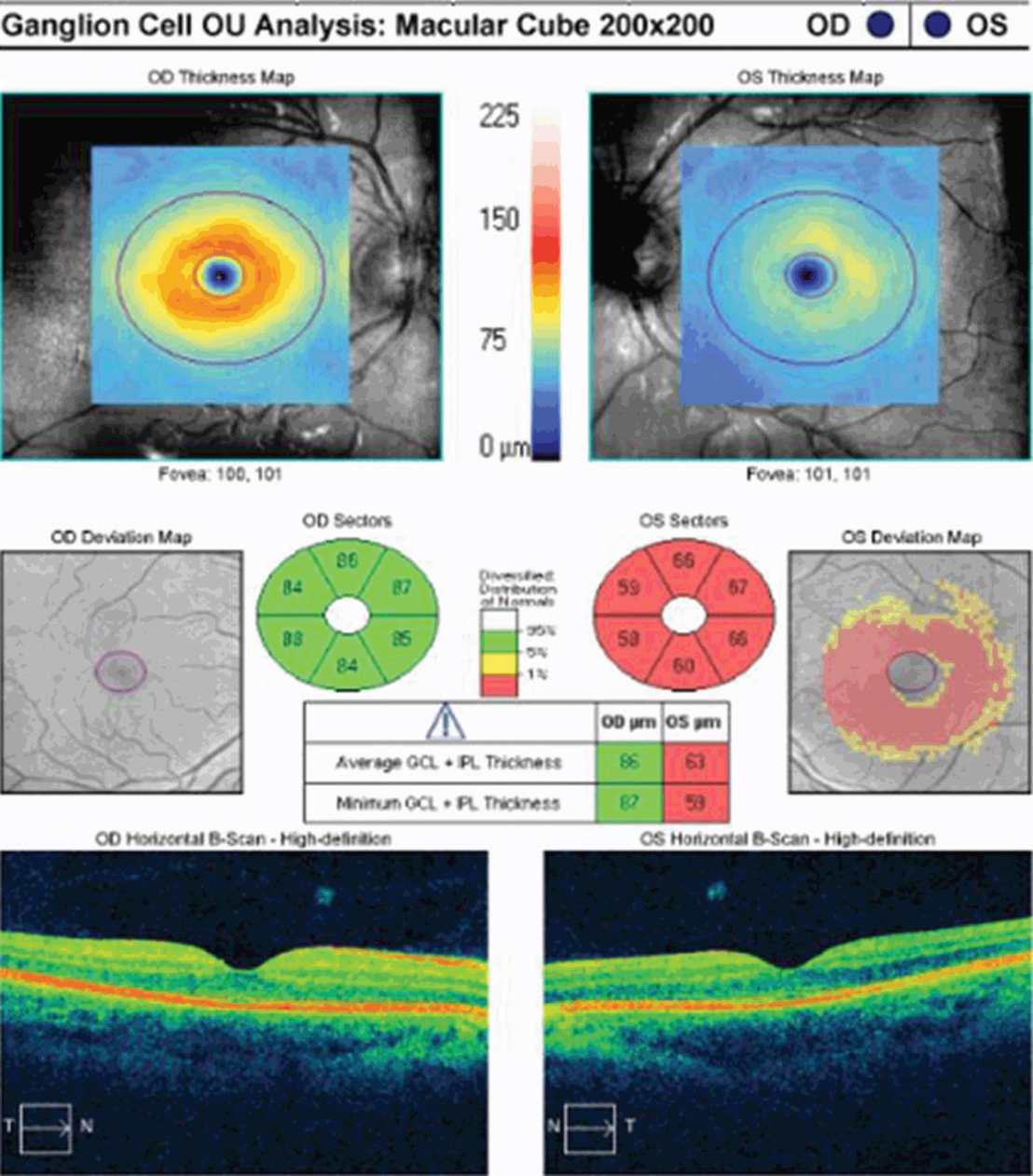
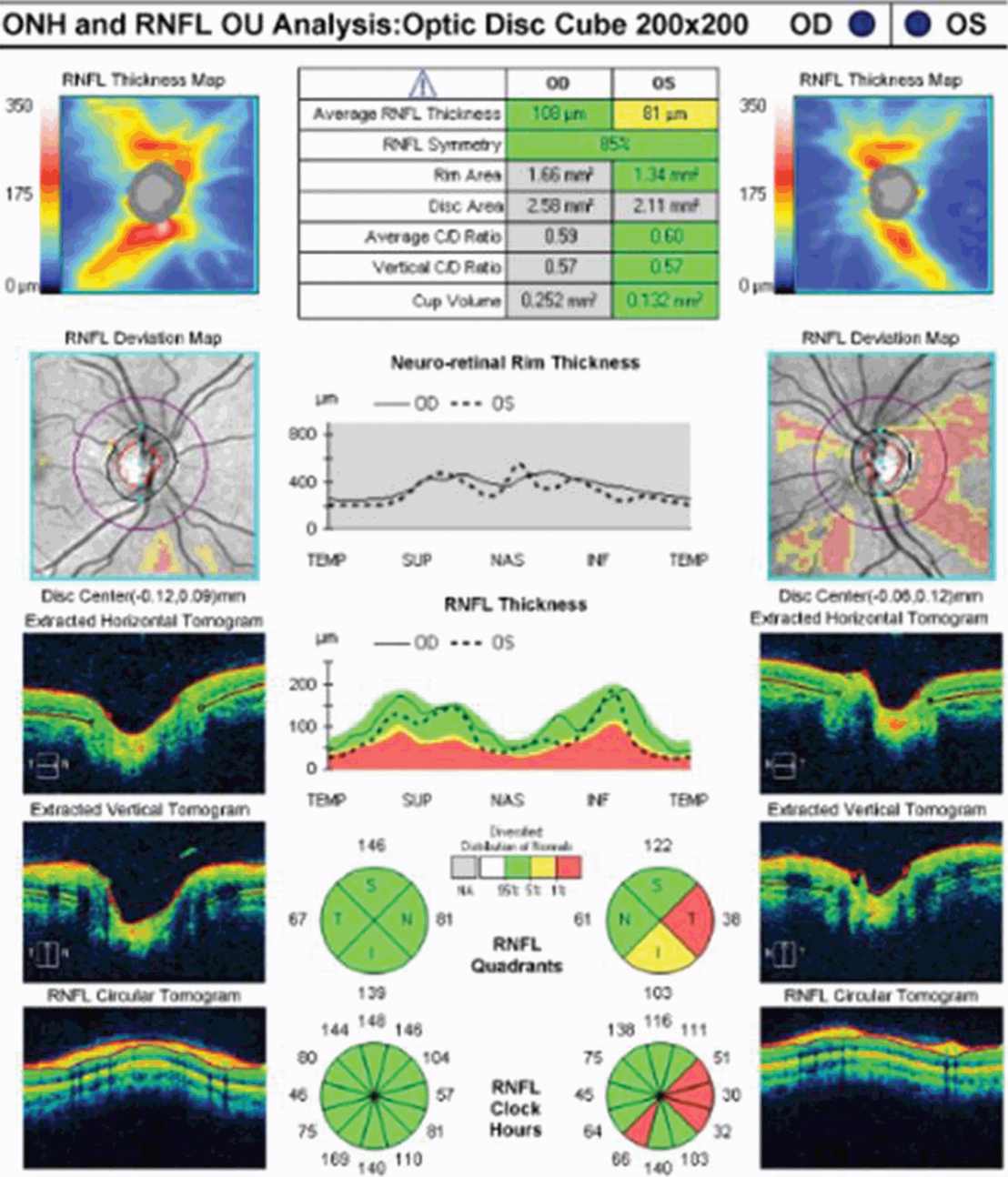
Interpreting RNFL changes in patients with recurrent ON
It can be difficult to detect RNFL changes in the setting of recurrent ON, because the extent of cumulative RNFL atrophy can be severe and the corresponding visual outcomes dire. In a recent OCT study of 193 MS patients, peripapillary RNFL values were compared between 29 eyes affected by two or more ON events, 125 eyes affected by a single ON event, and 232 eyes without ON. The mean RNFL values were significantly lower in recurrent ON eyes (64 μm) relative to single ON eyes (86 μm) and eyes without ON (100 μm) [14]. Yeh and colleagues [15] noted that average RNFL thickness decreased with an increasing number of ON episodes in pediatric patients with demyelinating syndromes, which supports the premise that recurrent inflammatory events have a cumulative impact in the AVP. This creates a practical challenge to using OCT in the diagnosis and management of acute ON in a patient with preexisting RNFL/MV/GCL+IPL atrophy, because increments of change in OCT measures decrease over time and may be subtle in the context of a new ON event.
Comparing RNFL changes in ON associated with neuromyelitis optica to RNFL changes in ON associated with MS
Neuromyelitis optica (NMO) is a severe inflammatory process of the optic nerves and spinal cord (with occasional brain involvement) that is generally associated with poor clinical recovery. Not surprisingly, RNFL atrophy tends to be extensive in ON eyes of patients with NMO. Several studies have explored the role of OCT in distinguishing ON associated with NMO from that associated with MS. Naismith [16] reported lower RNFL values in ON eyes of 22 NMO patients compared to ON eyes of 47 MS patients and noted that the superior and inferior RNFL quadrants were more severely affected in the former. In this study, the odds of falling into the NMO group increased by 8% for every 1-μm decrease in peripapillary RNFL thickness [16]. In a related study, there was significantly worse RNFL thinning in NMO ON eyes (64 μm) relative to RRMS ON eyes (88 μm) and control eyes (102 μm) [17]. Ratchford [17] reported that a first episode of ON was estimated to cause 24 μm more RNFL loss in NMO eyes relative to RRMS ON eyes. Nakamura [18] described lower RNFL values in NMO ON eyes than in MS ON eyes (64 μm versus 84 μm; p = 0.0006) and noted that the frequency of ON relapses and the time to initiate treatment with high-dose intravenous methylprednisolone significantly affected the preservation of RNFL thickness in NMO patients. Syc [11] evaluated 22 NMO patients and reported that GCL+ IPL thickness was decreased in NMO ON eyes compared to NMO non-ON eyes, and that NMO eyes with and without remote ON history had reduced GCL+ PIL thicknesses compared with those of healthy controls. The importance of diagnosing NMO early cannot be overstated because the natural history of ON associated with this disorder is poor and long-term immunosuppressive therapy is needed. It is noteworthy that patients who manifest an inter-eye asymmetry in RNFL thickness of 15 μm or greater have been deemed more likely to have the diagnosis of NMO (75%) than RRMS (24%) [17]. Yet in studies showing significantly more RNFL thinning in NMO ON eyes relative to MS ON eyes, there has been considerable overlap in the distribution of RNFL values. Hence, in using OCT to differentiate ON associated with NMO from ON associated with MS, it is important to be mindful of issues that could affect RNFL values in either group, including recurrent ON in the same eye and bilateral optic nerve involvement.
Optical coherence tomography and visual outcomes in acute optic neuritis
Optical coherence tomography has been viewed as a putative marker of neuronal and axonal integrity in the afferent visual pathway. If axonal and neuronal damage contribute to neurological disability, one would infer that OCT-measured changes in peripapillary RNFL, MV, and GCL + IPL should correspond to visual deficits in patients with acute ON. In this context, OCT would be a useful ancillary test that could be used to construct a structural–functional paradigm, by which factors influencing injury and repair might be better understood in MS patients.
High-Contrast Visual Acuity: High-Contrast Visual Acuity (HCVA) refers to the spatial resolving ability of the eye and has long been the mainstay of visual standard testing. The most common HCVA charts employed in clinical research studies include the Snellen and Early Treatment Diabetic Retinopathy Study (ETDRS) charts. The Snellen chart has letters of different sizes arranged from largest at the top to smallest at the bottom, which are read, one eye at a time, at a distance of six meters (20 feet). Snellen visual acuity is usually expressed as a fraction with the numerator equal to the distance from the chart and the denominator being the size of the smallest line that can be read. Because there are numerous limitations to Snellen visual acuity charts [2, 19], the ETDRS method has become the “gold standard” HCVA test for most current clinical trials. The ETDRS charts are superior to Snellen charts because interpatient differences are more accurately measured and longitudinal follow-up measurements have had more consistent precision, regardless of whether the patients had mild or severe vision loss. [19]. In the ETDRS charts, best corrected visual acuity can be converted to logMAR HCVA, which converts the geometric sequence of a traditional chart to a linear scale [19]. In logMAR notation, lower scores correspond to better vision, and as acuity becomes worse, the value of the logMAR increases.
In the setting of acute ON, HCVA impairment is generally maximal at onset, and the deficit in HCVA at one month can predict long-term recovery. Kupersmith and colleagues [20] used the ONTT database to evaluate various “cut points” for baseline and 1-month vision levels that predicted abnormal six-month vision. Based on their findings, failure to reach a one-month HCVA cut off of 20/50 correlated with having moderate-to-severe loss in this domain of function after six months [20]. On a practical level, if HCVA has not improved by one month, it should also prompt the physician to consider alternate diagnoses or ON mimics (Table 5.2).
At initial presentation, OCT-measured peripapillary RNFL values may be elevated, reflecting optic disc edema in patients with acute ON. In contrast, MV and GCL +IPL thickness maybe normal (in the case of a CIS patient), or perhaps slightly reduced (in the case of MS patients with preexisting damage to the AVP). Initially, there is generally poor concordance between OCT measures of structural integrity and functional outcomes in patients with acute ON. In the post-acute phase, however, lower RNFL, MV, and GCL + IPL values correlate with worse visual function [2, 7, 11–13]. In a prior prospective OCT study, measures of RNFL thickness were compared with logMAR HCVA scores for 54 patients observed a minimum of three months after acute ON [12]. Regression analyses showed that the predictive value for RNFL thickness was significant only for patients with RNFL measures less than 70 µm; lower RNFL values correlated with worse visual acuity outcomes after ON [12]. Not surprisingly, HCVA is worse in the post-acute phase of ON for NMO patients [17]. In their study of 26 NMO patients, Ratchford and colleagues [17] reported a threshold of RNFL thickness measuring 60 µm, below which visual acuity outcomes were poor in the setting of NMO.
Low-Contrast Letter Acuity: While HCVA has been used as the primary outcome in clinical trials in ophthalmology, it is a relatively crude measure of afferent visual function in MS. In fact, many patients will report significant vision problems after acute ON, even in the setting of 20/20 HCVA [21]. High-contrast visual acuity is determined under optimal circumstances of high contrast and high luminance, and it is therefore not surprising that HCVA tends to manifest the most obvious early improvement in acute ON patients. Yet the real visual world is one of varying spatial and temporal frequencies, contrast, color, luminance, and glare [2]. Over the past decade, Balcer and colleagues have spearheaded the implementation of low-contrast letter acuity (LCLA) testing in MS clinical research with the use of Sloan charts [22]. Sloan charts have a standardized format based on the ETDRS HCVA charts and employ three contrast levels: 100% (high-contrast), 2.5%, and 1.25% (lowest contrast level) [22]. Charts are scored letter by letter, and numbers of letters identified correctly constitute the score for each chart. Recently, visual improvement and loss by the LCLA chart has been defined as a seven-letter change in score [22]. In the IMPACT trial and in heterogeneous MS cohorts LCLA testing has been shown to be a highly reliable and practical means of identifying MS-related visual loss compared to other available tests [22]. Low-contrast letter acuity scores have also been shown to correlate with quality-of-life outcomes [22], and, in many respects, to capture the many shades of gray that represent visual perception in our day-to-day lives. In clinical practice, measuring LCLA can be hampered in acute ON patients who present with severe vision loss (worse than 20/200) because they will not be able to visualize the charts [21]. However, post-acutely, LCLA can be used to detect persistent visual deficits that may otherwise be missed in patients who have regained “normal” vision on standard HCVA and visual field testing. Previous studies have shown robust correlations between OCT-measured RNFL, MV, and LCLA outcomes in ON and MS patients [2, 7, 21]. In 2006, Fisher [21] conducted a cross-sectional study comparing RNFL thickness among MS eyes with a history of ON (MS ON eyes), MS eyes without a history of ON (MS non-ON eyes), and disease-free controls. They noted that RNFL thickness was reduced significantly among MS patients (92 µm) relative to controls (105 µm) and that RNFL values were particularly reduced in MS ON eyes (85 µm) [21]. Furthermore, for every one-line decrease in LCLA or contrast sensitivity score, the mean RNFL thickness decreased by 4 µm [21]. More recently, Syc [11] followed 20 acute ON patients with serial HCVA, LCLA, and OCT testing at baseline (within four weeks), three months, and six months of initial presentation. Low-contrast visual acuity at three months improved in ON eyes compared with baseline (2.5% p = 0.006; 1.25%, p = 0.02) [11]. In this same study, 22 NMO/ NMO-spectrum patients were studied in cross-sectional fashion. Ganglion cell layer + IPL, RNFL, and macular thickness values were decreased in NMO ON eyes compared to NMO non-ON eyes, and both NMO eyes with and without remote ON compared with those of healthy controls. Ganglion cell layer+ IPL thickness was significantly associated with 1.25% LCLA in NMO eyes, and 1.25% and 2.5% LCLA in NMO non-ON eyes [11]. Hence, LCLA testing is more sensitive in detecting visual deficits than HCVA testing in MS, and inclusion of these functional outcomes in the AVP model will better capture deficits that impact quality of life and day-to-day function in these patients.
Color Vision: Dyschromatopsia or loss of color vision is common in the setting of acute ON, and improves weeks to months after initial presentation. Indeed, the detection of disproportionate color vision loss can be key to making the diagnosis in patients with acute ON who manifest mild deficits in HCVA function. One of the challenges in interpreting color vision outcomes is that various modalities are used, and there is less standardization relative to HCVA, LCLA, and perimetry testing. Raz and colleagues [23] followed 21 patients with new-onset acute ON with serial HCVA, perimetry, color vision (Ishikawa pseudoisochromatic plates), visual evoked potentials (VEP), contrast sensitivity, motion perception, and OCT testing. In ON eyes, HCVA, visual field, and color perception were significantly impaired at initial presentation and recovered completely after one month [23]. Contrast sensitivity recovered after four months [23]. After 12 months, the RNFL values in both ON eyes and non-ON eyes were reduced when compared to the normal controls. The VEP amplitudes of ON eyes were decreased in the acute phase but not subsequently [23]. Both ON eyes and non-ON eyes of ON patients had significantly prolonged VEP latencies at all testing phases. This study demonstrated that functional recovery occurs at different time points, depending on the testing modality used to follow ON patients.
Various other testing techniques have been used effectively to capture color vision deficits in ON and MS patients. Hardy-Rand-Rittler (HRR) pseudo-isochromatic color plates have demonstrated an advantage over the Ishihara method, because the former are more sensitive to red–green and blue–yellow deficits caused by neuro-ophthalmic disorders [2]. Recently Villoslada and colleagues [24] studied 213 MS patient and 47 healthy controls to determine the relationship between HCVA, LCVA, color vision (HRR pseudoisochromatic plates and Lanthony D15 tests), and OCT measures in MS patients. Multiple sclerosis patients showed HCVA and LCLA deficits but exhibited even more profound abnormalities in color discrimination relative to controls [24]. Moura and colleagues [25] assessed chromatic discrimination in 35 MS patients (with and without ON) and 74 age-matched controls using the Cambridge color test (CCT) to determine the magnitude and chromatic axes of color vision loss in both patient groups. They also endeavored to evaluate age-related changes in chromatic discrimination in both patient groups compared to normal control subjects. Color thresholds for both ON eyes and non-ON eyes in MS patients were significantly higher than controls’ along the protan and tritan axes [25]. In addition, the ON and non-ON groups differed significantly along all three-color axes [25]. Multiple sclerosis patients manifested progressive color discrimination impairment with age (along the deutan and tritan axes) that was almost two times faster than controls, even in the absence of ON [25]. In a seminal OCT study, Trip [26] selected 25 ON patients in the post-acute phase with incomplete visual recovery, and performed cross-sectional analyses comparing OCT measured peripapillary RNFL thickness and MV, VEP, HCVA, perimetry, and color vision testing (Farnsworth–Munsell). In this study, RNFL thickness was reduced by 33%, and macular volume by 11% in ON eyes relative to control eyes [26]. Interestingly, in ON eyes lower MV values were associated with color vision loss. The authors highlighted that axons originating in the macula pass in the papillomacular bundle to the temporal side of the optic disc; consistent with this, MV values were related to the thickness of the temporal RNFL quadrant in this study [26].
Given that NMO patients have generally more severe ON than that seen in MS patients, RNFL values are reduced in NMO ON eyes, and they correlate with more severe color vision deficits than their MS counterparts. In a cross-sectional study of 15 NMO patients, 15 MS patients, and 23 controls, Merle and colleagues [27] reported lower RNFL values in NMO patients (65 µm) relative to MS (84 µm) and control (106 µm) eyes. This group employed OCT, HCVA, LCLA (1.25% and 2.5%), perimetry, and Farnsworth 100 Hue color vision testing. There was a significant correlation between the average peripapillary RNFL thickness, HCVA, LCLA, and visual field scores [27]. Yet, there was no correlation between OCT measures and color vision testing. These investigators did not use temporal RNFL or MV measurements in their study, which may in part explain the differences in their results as compared to the findings by Trip et al. [27]. Future studies could further explore the optimal color vision test to use in patients with acute ON, and determine whether GCL+ IPL thickness as a marker of neuronal integrity correlates with color vision deficits in various phases of recovery.
Visual Field Testing: Visual field testing has been described as the “cornerstone” of the sensory visual examination and provides invaluable information about the integrity of afferent visual pathway function from the retina to the visual cortex [28]. Perimetry has evolved through stages since original confrontation-based techniques to allow quantification and statistical analysis in its currently used computerized forms. This provides critical information about visual function, including both central and peripheral channels. Moreover, many of the perimeters in common use today are readily available in most cities around the developed world, allowing for standardization between centers, and easier comparisons across offices over time. In the recent 15-year follow-up from the ONTT, Keltner [29] defined visual field characteristics and classifications for the entire cohort, from baseline through 15 years (10,443 visual fields). At initial presentation, 100% of the visual fields from the ON eyes and 75% of the visual fields from the fellow (non-ON) eyes in patients were abnormal [29]. After year one of the study, 50% of the visual fields were abnormal in ON eyes, whereas the abnormal visual field frequency in non-ON eyes ranged between 34% and 40% [29]. Diffuse and central visual field losses were more prominent in ON eyes than non-ON eyes at baseline. Retinal nerve fiber bundle defects (partial arcuate, paracentral, and arcuate) were the most prominent localized abnormalities in ON and non-ON eyes during the study [29]. After 15 years, 40% of abnormalities in the ON eyes and 26% in the non-ON eyes consisted of localized defects [29]. Foveal threshold, as measured by Humphrey perimetry, correlated with HCVA and contrast sensitivity in ON eyes at baseline, six months, and one year [29].
For all of the established advantages of automated perimetry, there are potential pitfalls with this testing modality in a patient population that is subject to fatigue-related visual dysfunction. Wall and colleagues [30] followed 17 patients with ON and ten healthy control subjects with repeat intra-day and interday automated perimetry testing (five Humphrey 30–2 tests were administered during a seven-hour period on the same day and at the same period on five separate days). Optic neuritis patients demonstrated variations in visual field sensitivity outside the entire range of variability for normal controls [30]. These variations occurred for multiple tests performed on the same day, at specific times, and for tests performed at specific times on different days [30]. Thus, when using automated perimetry, or for that matter any subjective psychophysical test to follow ON and MS patients, distinguishing true change from variability remains a challenge. In the case of automated perimetry, this requires more than comparing a current visual field test with the most recent previous visual field test; rather, trends should be observed over time.
In 2006, our group followed 54 patients with acute ON prospectively over a 12-month period with serial OCT, HCVA, and automated perimetry testing [12]. Thinning of the RNFL was seen in the majority of patients (74%), and it tended to occur within 3 to 6 months of symptom onset [12]. The average peripapillary RNFL values were thinner in ON eyes (78 µm) than in non-ON eyes (100 µm) [12]. Patients with incomplete visual recovery demonstrated worse RNFL loss after ON. Regression analyses revealed a threshold of RNFL thickness (75 µm), below which RNFL measurements predicted persistent visual field dysfunction [12]. In this same study, measures of RNFL thickness were compared with log MAR visual acuity for 54 patients observed a minimum of three months after acute ON. The predictive value for RNFL thickness was significant only for patients with measures less than 70 µm, below which RNFL values were associated with worse HCVA outcomes after ON [12]. Interestingly, in subsequent work, an OCT-measured RNFL threshold of 80 µm was found to distinguish patients with persistent LCLA deficits after acute ON [22]. Given that LCLA, automated perimetry techniques, and HCVA are variably sensitive to persistent visual deficits after acute ON, OCT testing may reveal a range of RNFL (and MV and GCL+IPL) thresholds that predict visual recovery, depending on visual outcome used.
Visual Evoked Potential Testing: The VEP is a response of the brain to repeated visual stimulation and has traditionally been recorded when visual field is stimulated with a single checkerboard pattern in the full-field (ff-VEP). The VEP is known to be generated at the level of striate cortex. The magnitude of the VEP is interpreted to reflect the number of functional afferent fibers reaching this region [31, 32]. In ON patients, the number of functional afferent fibers is believed to be determined by the severity of the inflammation and axonal degeneration along the visual pathway [31, 32]. Therefore, diminished VEP amplitude indicates inflammation-induced conduction block, axonal atrophy, or a combination of both [31, 32]. Subsequently, an increase in VEP amplitude is a consequence of resumed conduction in previously blocked fibers, due to resolution of inflammation and edema, or possibly expansion of synaptic activity along the visual pathway [31, 32]. Delayed VEP conduction is recognized as one of the earliest features of acute ON, with the subsequent shortening of latency thought to represent remyelination [31, 32]. Because it is a summation of a large number of neuronal elements, the ffVEP is greatly dominated by the macular region due to its cortical overrepresentation [31, 32]. Moreover, the waveform of the ffVEP is prone to cancellation and distortion, which sometimes leads to apparent, rather than real, latency delay [31, 32]. In contrast, the multifocal VEP (mfVEP) allows stimulation of small areas of the visual field. The result is a detailed topographical assessment of small groups of axons within the optic nerve and visual cortex, which is resistant to waveform distortion [31, 32]. In a recent study, Klistorner and colleagues [31] used mfVEP and OCT testing to study 25 subjects with acute unilateral ON. While mfVEP amplitude asymmetry at baseline varied significantly among the patients, it was, on average, very high, indicating considerable reduction of amplitude in ON eyes [31]. The inter-eye asymmetry in mfVEP amplitude decreased over time, indicating functional recovery [31]. There was a negative correlation between the inter-eye asymmetry of OCT-measured RNFL thickness and that of mfVEP amplitude at one month, consistent with vasogenic edema in the acute phase (causing an increase in RNFL thickness with a corresponding reduction in mfVEP amplitude) [31]. Over the course of recovery, the correlation became progressively more robust, suggesting the diminishing role of optic nerve edema in measured RNFL thickness and unmasking the association between RNFL atrophy and low mfVEP amplitude [31]. The potential correlation between OCT-measured RNFL values and mfVEP measures of anterior visual pathway damage was demonstrated by the same group, who evaluated 32 patients with unilateral ON and 25 control subjects with mfVEP and OCT testing [32]. The mean RNFL thickness in ON eyes (85 μm) was reduced by 19% compared with control eyes (104 μm). There was a 40% reduction in the amplitude of the mfVEP in ON eyes relative to control eyes [32]. In addition to demonstrating the utility of mfVEP in tracking optic nerve injury in ON patients, this study further confirmed the significant correlations between structural and functional measures of optic nerve integrity, and showed that demyelination contributes to axonal loss. It may, therefore, be feasible to pair mfVEP and OCT testing to capture the synergistic effects of acute demyelination and axonal loss over time in ON/MS patients. Furthermore, the putative relationship between the VEP latency and axonal loss encourages the notion that therapeutic interventions aimed at reducing the effects of demyelination or enhancing remyelination may be given a trial in the AVP model.
Motion Perception Testing: Standard tests of visual function generally interrogate our perception of “form,” and tend to ignore perception of motion, which has an enormous impact on the day-to-day function. In the convalescent phase, many ON patients report the “Pulfrich phenomenon” (a binocular perception that a small target moving in a frontal plane is traveling in an elliptical path) and describe difficulties tracking moving objects. The hypothesis that ON can have prolonged effects on visual motion processing, which may persist after there has been an objective return to normal “form” perception, was further explored by Raz and colleagues in a series of elegant papers [23, 33]. They prospectively followed 21 ON patients over one year with tests of spatial and dynamic visual function including: HCVA, perimetry, contrast sensitivity, color vision, VEP, and OCT testing [23, 33]. In addition, the authors developed a novel set of motion perceptual tasks to test dynamic visual deficits as part of their protocol [23, 33]. In ON eyes, visual acuity, visual field, and color vision deficits were significantly impaired in the acute phase, and subsequently improved after one month [23]. Contrast sensitivity deficits tended to persist somewhat longer, improving four months after symptom onset [23]. As compared to tests of spatial visual function, motion perception was impaired during the full follow-up period of one year [23]. Thus, motion perception testing revealed the most significant and prolonged impairment after ON and was independent of contrast sensitivity levels [23].
In a follow-up study, the same group aimed to identify mechanisms underpinning the sustained deficits in dynamic visual functions following ON. They hypothesized that motion perception may be more vulnerable to slowed conduction in the optic nerve, which could be measured with VEP testing [33]. To explore this theory further, they did serial motion perception and ffVEP testing at presentation, one month, four months, and twelve months after acute ON [33]. The VEP amplitudes in ON eyes were significantly reduced compared to fellow eyes in the acute phase, but these differences resolved in later phases of recovery [33]. As has been previously reported, visual performance with HCVA, contrast sensitivity, and motion perception at one month was highly predictive of visual recovery a year after acute ON [33]. Intact VEP amplitudes were associated with recovered visual acuity and contrast sensitivity after ON, suggesting that these visual functions depend on a sufficient amount of visual information reaching the cortex [33]. Yet motion perception was impaired even in patients with intact VEP amplitudes, indicating that an intact amount of visual projection alone does not impact dynamic visual function [33]. Instead, while the magnitude of contrast sensitivity improvement related to the extent of VEP amplitude restoration, the magnitude of motion perception improvement depended on the extent of VEP latency reduction post-ON [33]. From these findings, it was inferred that there is a need for rapid transmission of visual input to perceive motion. Moreover, motion perception testing in concert with VEP may serve to assess the processes of demyelination and remyelination in the AVP model of MS.
Binocular Summation: Binocular summation refers to the improved visual function observed when patients perform threshold tasks such as contrast detection with a binocular view as compared to monocular vision [34]. Conversely, binocular inhibition refers to worse outcomes for visual threshold tasks obtained with binocular vision as compared to the monocular view obtained when patients use their better seeing eye [34]. The phenomena of binocular summation and inhibition are not well understood, but appear to be related to neural input from both eyes within the post-geniculate visual pathway [34]. Given that binocular summation is most likely processed at the cortical level, MS and ON patients may experience functional limitations due to interruptions in normal cortical signaling. In a recent study of 1,007 patients with MS and 324 disease-free controls, binocular summation was substantial for LCLA at the 2.5% and 1.25% levels [34]. With HCVA, only 3% of patients showed similar degrees of summation [34]. Increasing age, greater interocular differences in acuity, and prior ON were factors associated with lower magnitudes of binocular summation and worse binocular inhibition [34]. Greater degrees of binocular summation were predictive of better quality-of-life outcomes, indicating that the capacity to use both eyes together is an important factor in determining how well patients with MS perform daily activities [34]. In future studies, the effects of binocular summation and inhibition could potentially be compared to OCT (as a structural measure of neuronal and axonal damage), VEP (as a demyelination and remyelination marker), and functional MRI (as a means of detecting cortical activation). In this way, the relative contribution of cortical compensation to visual recovery, and the factors that govern the potential for cortical plasticity, may be determined after acute ON in patients with CNS demyelinating disorders.
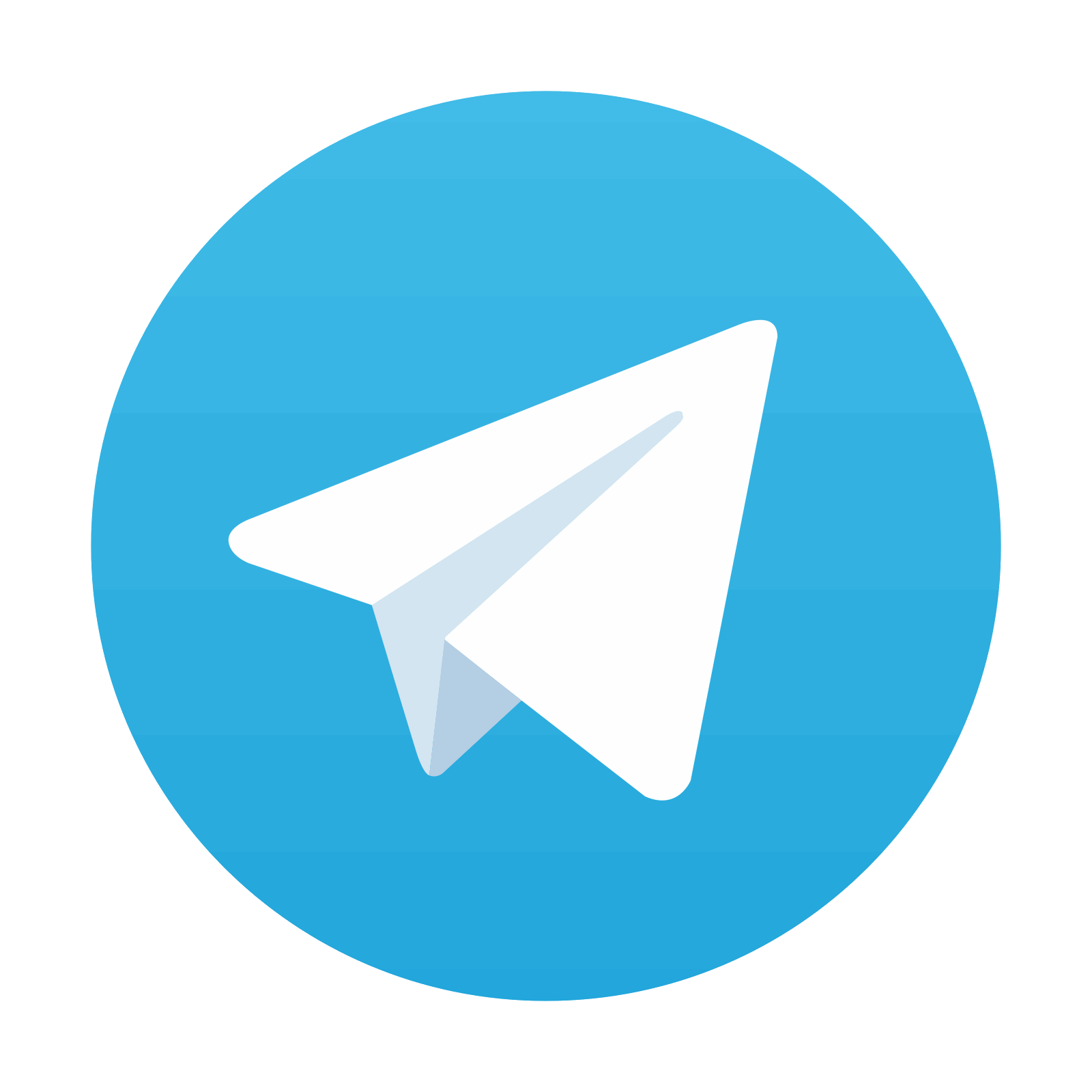
Stay updated, free articles. Join our Telegram channel

Full access? Get Clinical Tree
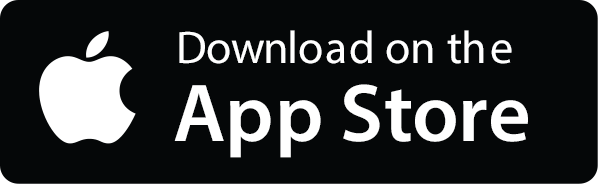
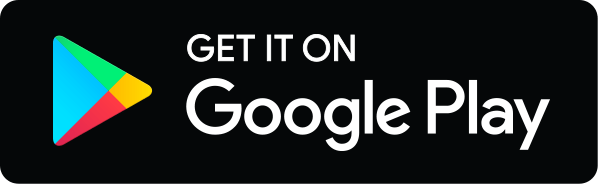